Reversed-phase liquid chromatography (RP-LC) is a mode of liquid chromatography in which non-polar stationary phase and polar mobile phases are used for the separation of organic compounds.[1][2][3] The vast majority of separations and analyses using high-performance liquid chromatography (HPLC) in recent years are done using the reversed phase mode. In the reversed phase mode, the sample components are retained in the system the more hydrophobic they are.[4]
The factors affecting the retention and separation of solutes in the reversed phase chromatographic system are as follows:
a. The chemical nature of the stationary phase, i.e., the ligands bonded on its surface, as well as their bonding density, namely the extent of their coverage.
b. The composition of the mobile phase. Type of the bulk solvents whose mixtures affect the polarity of the mobile phase, hence the name modifier for a solvent added to affect the polarity of the mobile phase.
C. Additives, such as buffers, affect the pH of the mobile phase, which affect the ionization state of the solutes and their polarity.
In order to retain the organic components in mixtures, the stationary phases, packed within columns, consist of a hydrophobic substrates, bonded to the surface of porous silica-gel particles in various geometries (spheric, irregular), at different diameters (sub-2, 3, 5, 7, 10 um), with varying pore diameters (60, 100, 150, 300, A). The particle's surface is covered by chemically bonded hydrocarbons, such as C3, C4, C8, C18 and more. The longer the hydrocarbon associated with the stationary phase, the longer the sample components will be retained. Some stationary phases are also made of hydrophobic polymeric particles, or hybridized silica-organic groups particles, for method in which mobile phases at extreme pH are used. Most current methods of separation of biomedical materials use C-18 columns, sometimes called by trade names, such as ODS (octadecylsilane) or RP-18.
The mobile phases are mixtures of water and polar organic solvents, the vast majority of which are methanol and acetonitrile. These mixtures usually contain various additives such as buffers (acetate, phosphate, citrate), surfactants (alkyl amines or alkyl sulfonates) and special additives (EDTA). The goal of using supplements of one kind or another is to increase efficiency, selectivity, and control solute retention.
Stationary phases
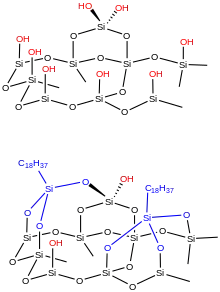
The history and evolution of reversed phase stationary phases in described in detail in an article by Majors, Dolan, Carr and Snyder.[6]
In the 1970s, most liquid chromatography runs were performed using solid particles as the stationary phases, made of unmodified silica gel or alumina. This type of technique is now referred to as normal-phase chromatography. Since the stationary phase is hydrophilic in this technique, and the mobile phase were a-polar, consisted of non-polar organic solvents such as hexane and heptane, biomolecules with hydrophilic properties in the sample had high affinity to the stationary phase, therefore they adsorb to it strongly. Moreover, they were not dissolved easily in the mobile phase solvents. At the same time hydrophobic molecules experience less affinity to the polar stationary phase, and elute through it early with not enough retention. This was the reasons why during the 1970s the silica based particles were treated with hydrocarbons, immobilized or bonded on their surface, and the mobile phases were switched to aqueous and polar in nature, to accommodate biomedical substances.
The use of a hydrophobic stationary phase and polar mobile phases is essentially the reverse of normal phase chromatography, since the polarity of the mobile and stationary phases have been inverted – hence the term reversed-phase chromatography.[7][8] As a result, hydrophobic molecules in the polar mobile phase tend to adsorb to the hydrophobic stationary phase, and hydrophilic molecules in the sample pass through the column and are eluted first.[7][9] Hydrophobic molecules can be eluted from the column by decreasing the polarity of the mobile phase using an organic (non-polar) solvent, which reduces hydrophobic interactions. The more hydrophobic the molecule, the more strongly it will bind to the stationary phase, and the higher the concentration of organic solvent that will be required to elute the molecule.
Many of the mathematical parameters of the theory of chromatography and experimental considerations used in other chromatographic methods apply to RP-LC as well (for example, the selectivity factor, chromatographic resolution, plate count, etc. It can be used for the separation of a wide variety of molecules. It is typically used for separation of proteins,[10] because the organic solvents used in normal-phase chromatography can denature many proteins.
Today, RP-LC is a frequently used analytical technique. There are huge variety of stationary phases available for use in RP-LC, allowing great flexibility in the development of the separation methods.[11][12]
Silica-based stationary phases
Silica gel particles are commonly used as a stationary phase in high-performance liquid chromatography (HPLC) for several reasons,[13][14] including:
- High surface area: Silica gel particles have a high surface area, allowing direct interactions with solutes or after bonding of variety of ligands for versatile interactions with the sample molecules, leading to better separations.
- Chemical and thermal stability and inertness:[15] Silica gel is chemically stable, as it usually does not react with either the solvents of the mobile phase nor the compounds being separated, resulting in accurate, repeatable and reliable analyses.
- Wide applicability:[16] Silica gel is versatile and can be modified with various functional groups, making it suitable for a wide range of analytes and applications.
- Efficient separation: The unique properties of silica gel particles, combined with their high surface area and controlled average particle diameter pore size,[17] facilitate efficient and precise separation of compounds in HPLC.
- Reproducibility: Silica gel particles can offer high batch-to-batch reproducibility, which is crucial for consistent and reliable HPLC analyses throughout decades.
- Particle diameter and pore size control:[18][19] Silica gel can be engineered to have specific pore sizes, enabling precise control over separation based on molecular size.
- Cost-effectiveness: Silica is the most abundant element on earth, hence its gel is a cost-effective choice for HPLC applications, making it widely adopted in laboratories.
The United States Pharmacopoeia (USP) has classified HPLC columns by L# types.[20] The most popular column in this classification is an octadecyl carbon chain (C18)-bonded silica (USP classification L1).[21] This is followed by C8-bonded silica (L7), pure silica (L3), cyano-bonded silica (CN) (L10) and phenyl-bonded silica (L11). Note that C18, C8 and phenyl are dedicated reversed-phase stationary phases, while CN columns can be used in a reversed-phase mode depending on analyte and mobile phase conditions. Not all C18 columns have identical retention properties. Surface functionalization of silica can be performed in a monomeric or a polymeric reaction with different short-chain organosilanes used in a second step to cover remaining silanol groups (end-capping). While the overall retention mechanism remains the same, subtle differences in the surface chemistries of different stationary phases will lead to changes in selectivity.
Modern columns have different polarity depending on the ligand bonded to the stationary phase. PFP is pentafluorphenyl. CN is cyano. NH2 is amino. ODS is octadecyl or C18. ODCN is a mixed mode column consisting of C18 and nitrile.[22]
Recent developments in chromatographic supports and instrumentation for liquid chromatography (LC) facilitate rapid and highly efficient separations, using various stationary phases geometries.[23] Various analytical strategies have been proposed, such as the use of silica-based monolithic supports, elevated mobile phase temperatures, and columns packed with sub-3 μm superficially porous particles (fused or solid core)[24] or with sub-2 μm fully porous particles for use in ultra-high-pressure LC systems (UHPLC).[25]
Mobile phases
A comprehensive article on the modern trends and best practices of mobile phase selection in reversed-phase chromatography was published by Boyes and Dong.[26] A mobile phase in reversed-phase chromatograpy consists of mixtures of water or aqueous buffers, to which organic solvents are added, to elute analytes from a reversed-phase column in a selective manner.[7][27] The added organic solvents must be miscible with water, and the two most common organic solvents used are acetonitrile and methanol. Other solvents can also be used such as ethanol or 2-propanol (isopropyl alcohol) and tetrahydrofuran (THF). The organic solvent is called also a modifier, since it is added to the aqueous solution in the mobile phase in order to modify the polarity of the mobile phase. Water is the most polar solvent in the reversed phase mobile phase; therefore, lowering the polarity of the mobile phase by adding modifiers enhances its elution strength. The two most widely used organic modifiers are acetonitrile and methanol, although acetonitrile is the more popular choice. Isopropanol (2-propanol) can also be used, because of its strong eluting properties, but its use is limited by its high viscosity, which results in higher backpressures. Both acetonitrile and methanol are less viscous than isopropanol, although a mixture of 50:50 percent of methanol:water is also very viscous and causes high backpressures.
All three solvents are essentially UV transparent. This is a crucial property for common reversed phase chromatography since sample components are typically detected by UV detectors. Acetonitrile is more transparent than the others in low UV wavelengths range, therefore it is used almost exclusively when separating molecules with weak or no chromophores (UV-VIS absorbing groups), such as peptides. Most peptides only absorb at low wavelengths in the ultra-violet spectrum (typically less than 225 nm) and acetonitrile provides much lower background absorbance at low wavelengths than the other common solvents.
The pH of the mobile phase can have an important role on the retention of an analyte and can change the selectivity of certain analytes.[28][29] For samples containing solutes with ionized functional groups, such as amines, carboxyls, phosphates, phosphonates, sulfates, and sulfonates, the ionization of these groups can be controlled using mobile phase buffers.[30]
For example, carboxylic groups in solutes become increasingly negatively charged as the pH of the mobile phase rises above their pKa, hence the whole molecule becomes more polar and less retained on the a-polar stationary phase. In this case, raising the pH of the phase mobile above 4–5 = pH (which is the typical pKa range for carboxylic groups) increases their ionization, hence decreases their retention. Conversely, using a mobile phase at a pH lower than 4[31] will increase their retention, because it will decrease their ionization degree, rendering them less polar.
The same considerations apply to substances containing basic functional groups, such as amines, whose pKa ranges are around 8 and above, are retained more, as the pH of the mobile phase increases, approaching 8 and above, because they are less ionized, hence less polar. However, in the case of high pH mobile phases, most of the traditional silica gel based Reversed Phase columns are generally limited for use with mobile phases at pH 8 and above, therefore, control over the retention of amines in this range is limited.[32]
The choice of buffer type is an important factor in RP-LC method development, as it can affect the retention, selectivity, and resolution of the analytes of interest.[26] When selecting a buffer for RP-HPLC, there are a number of factors to consider, including:
- The desired pH of the mobile phase: Buffers are most effective around their pKa value, so it is important to choose a buffer with a pKa that is close to the desired mobile phase pH needed.
- The solubility of the buffer in the organic solvent: The buffer must be compatible with the organic solvent that is being used in the mobile phase, mostly with the common organic solvents mentioned above, acetonitrile, methanol, and isopropanol.
- The UV cut-off of the buffer: In case of UV detection, the buffer should have a UV absorption that is below the detection wavelength of the analytes of interest. This will prevent the buffer from interfering with the detection of that analytes.
- The compatibility of the buffer with the detector: If mass spectrometry (MS) is being used for detection, the buffer must be compatible with the mass spectrometry (MS) instrument.[33] Some buffers, such as those containing phosphate salts, cannot be used with the MS detectors, as they are not volatile as needed, and they interfere with the MS detection by suppressing the analytes ionization, making them undetected by MS.
Some of the most common buffers used in RP-HPLC include:[34]
- Phosphate buffers: Phosphate buffer is versatile and can be used to achieve a wide range of pH values, thanks to 3 pKa values. They also have very low UV background for UV detection. However, they are not appropriate for MS detection.
- Acetate buffers: Acetate buffers are also versatile and can be used to achieve range of pH values typically used in RP-LC. In terms of UV detection at sub 220 nm wavelength, it is not so favorable. The ammonium acetate buffer is compatible with MS.
- Formate buffers: Formate buffers is similar to the acetate buffer in terms of range of pHs used and limited UV detection under 225 nm. Its ammonium acetate is also compatible with MS.
- Ammonium buffers: Ammonium buffers are volatile and are often used in LC-MS methods. They also are limited for low UV detection.
Charged analytes can be separated on a reversed-phase column by the use of ion-pairing (also called ion-interaction). This technique is known as reversed-phase ion-pairing chromatography.[35]
Elution can be performed isocratically (the water-solvent composition does not change during the separation process) or by using a solution gradient (the water-solvent composition changes during the separation process, usually by decreasing the polarity).
See also
References
- ↑ IUPAC, Compendium of Chemical Terminology, 2nd ed. (the "Gold Book") (1997). Online corrected version: (2006–) "reversed-phase chromatography". doi:10.1351/goldbook.R05376
- ↑ Žuvela, Petar; Skoczylas, Magdalena; Jay Liu, J.; Ba̧Czek, Tomasz; Kaliszan, Roman; Wong, Ming Wah; Buszewski, Bogusław; Héberger, K. (2019). "Column Characterization and Selection Systems in Reversed-Phase High-Performance Liquid Chromatography". Chemical Reviews. 119 (6): 3674–3729. doi:10.1021/acs.chemrev.8b00246. PMID 30604951. S2CID 58631771.
- ↑ Dorsey, John G.; Dill, Ken A. (1989). "The molecular mechanism of retention in reversed-phase liquid chromatography". Chemical Reviews. 89 (2): 331–346. doi:10.1021/cr00092a005.
- ↑ Ganesh, V.; Poorna Basuri, P.; Sahini, K.; Nalini, C.N. (2023). "Retention behaviour of analytes in reversed‐phase high‐performance liquid chromatography—A review". Biomedical Chromatography. 37 (7): e5482. doi:10.1002/bmc.5482. ISSN 0269-3879. PMID 35962484. S2CID 251540223.
- ↑ Pape, Peter G. (2017). "Silylating Agents". Kirk-Othmer Encyclopedia of Chemical Technology. pp. 1–15. doi:10.1002/0471238961.1909122516011605.a01.pub3. ISBN 9780471238966.
- ↑ Majors, Ronald; Dolan, John; Carr, Peter; Snyder, Lloyd (2010). "New Horizons in Reversed-Phase Chromatography". LCGC North America. LCGC North America-06-01-2010. 28 (6): 418–430.
- 1 2 3 Akul Mehta (December 27, 2012). "Principle of Reversed-Phase Chromatography HPLC/UPLC (with Animation)". PharmaXChange. Retrieved 10 January 2013.
- ↑ I Molnár and C Horváth (September 1976). "Reverse-phase chromatography of polar biological substances: separation of catechol compounds by high-performance liquid chromatography". Clinical Chemistry. 22 (9): 1497–1502. doi:10.1093/clinchem/22.9.1497. PMID 8221. Retrieved 10 January 2013.
- ↑ (Clinical Biochemistry, T.W.Hrubey, 54)
- ↑ Evans, David R.H.; Romero, Jonathan K.; Westoby, Matthew (2009), "Chapter 9 Concentration of Proteins and Removal of Solutes", Guide to Protein Purification, 2nd Edition, Methods in Enzymology, vol. 463, Elsevier, pp. 97–120, doi:10.1016/s0076-6879(09)63009-3, ISBN 978-0-12-374536-1, PMID 19892169, retrieved 2022-10-13
- ↑ Žuvela, Petar; Skoczylas, Magdalena; Jay Liu, J.; Ba̧czek, Tomasz; Kaliszan, Roman; Wong, Ming Wah; Buszewski, Bogusław (2019). "Column Characterization and Selection Systems in Reversed-Phase High-Performance Liquid Chromatography". Chemical Reviews. 119 (6): 3674–3729. doi:10.1021/acs.chemrev.8b00246. ISSN 0009-2665. PMID 30604951. S2CID 58631771.
- ↑ Rusli, Handajaya; Putri, Rindia M.; Alni, Anita (2022). "Recent Developments of Liquid Chromatography Stationary Phases for Compound Separation: From Proteins to Small Organic Compounds". Molecules. 27 (3): 907. doi:10.3390/molecules27030907. ISSN 1420-3049. PMC 8840574. PMID 35164170.
- ↑ "Advantages of Silica Gels for HPLC Packing Applications - AGC Chemicals". 2020-11-10. Retrieved 2023-10-17.
- ↑ Qiu, Hongdeng; Liang, Xiaojing; Sun, Min; Jiang, Shengxiang (2011). "Development of silica-based stationary phases for high-performance liquid chromatography". Analytical and Bioanalytical Chemistry. 399 (10): 3307–3322. doi:10.1007/s00216-010-4611-x. ISSN 1618-2650. PMID 21221544. S2CID 40721088.
- ↑ Claessens, H. A.; van Straten, M. A. (2004). "Review on the chemical and thermal stability of stationary phases for reversed-phase liquid chromatography". Journal of Chromatography A. The Stationary Phase and Chromatographic Retention: Honoring J.J. Kirkland. 1060 (1): 23–41. doi:10.1016/j.chroma.2004.08.098. ISSN 0021-9673.
- ↑ Žuvela, Petar; Skoczylas, Magdalena; Jay Liu, J.; Ba̧czek, Tomasz; Kaliszan, Roman; Wong, Ming Wah; Buszewski, Bogusław (2019). "Column Characterization and Selection Systems in Reversed-Phase High-Performance Liquid Chromatography". Chemical Reviews. 119 (6): 3674–3729. doi:10.1021/acs.chemrev.8b00246. ISSN 0009-2665. PMID 30604951. S2CID 58631771.
- ↑ Cheong, Won Jo (2014). "Porous Silica Particles As Chromatographic Separation Media: A Review". Bulletin of the Korean Chemical Society. 35 (12): 3465–3474. doi:10.5012/bkcs.2014.35.12.3465. ISSN 0253-2964.
- ↑ "HPLC Column - Pore Sizes and Particle Diameters". www.crawfordscientific.com. Retrieved 2023-10-17.
- ↑ "Pore Size vs. Particle Size in HPLC Columns". Chrom Tech, Inc. Retrieved 2023-10-17.
- ↑ "USP L Column Listing - HPLC Columns & Bulk Media". SMT. Retrieved 2023-10-17.
- ↑ USP Chromatographic Reagents 2007-2008: Used in USP-NF and Pharmacopeial Forum. United States Pharmacopeia. 2007.
- ↑ Žuvela, Petar; Skoczylas, Magdalena; Jay Liu, J.; Ba̧czek, Tomasz; Kaliszan, Roman; Wong, Ming Wah; Buszewski, Bogusław (2019-03-27). "Column Characterization and Selection Systems in Reversed-Phase High-Performance Liquid Chromatography". Chemical Reviews. 119 (6): 3674–3729. doi:10.1021/acs.chemrev.8b00246. ISSN 0009-2665. PMID 30604951. S2CID 58631771.
- ↑ Guillarme, Davy; Ruta, Josephine; Rudaz, Serge; Veuthey, Jean-Luc (2010). "New trends in fast and high-resolution liquid chromatography: a critical comparison of existing approaches". Analytical and Bioanalytical Chemistry. 397 (3): 1069–1082. doi:10.1007/s00216-009-3305-8. ISSN 1618-2642. PMID 19998028. S2CID 21218108.
- ↑ Fekete, Szabolcs; Olh, Erzsbet; Fekete, Jen (2012). "Fast liquid chromatography: The domination of core?shell and very fine particles". Journal of Chromatography A. 1228: 57–71. doi:10.1016/j.chroma.2011.09.050. PMID 21982449.
- ↑ Fekete, Szabolcs; Ganzler, Katalin; Fekete, Jenő (2011). "Efficiency of the new sub-2μm core–shell (Kinetex™) column in practice, applied for small and large molecule separation". Journal of Pharmaceutical and Biomedical Analysis. 54 (3): 482–490. doi:10.1016/j.jpba.2010.09.021. PMID 20940092.
- 1 2 Boyes, Barry; Dong, Michael (2018). "Modern Trends and Best Practices in Mobile-Phase Selection in Reversed-Phase Chromatography". LCGC North America. LCGC North America-10-01-2018. 36 (10): 752–768.
- ↑ Boyes, Barry; Dong, Michael (2018). "Modern Trends and Best Practices in Mobile-Phase Selection in Reversed-Phase Chromatography". LCGC North America. LCGC North America-10-01-2018. 36 (10): 752–768.
- ↑ Heyrman, Aimee N.; Henry, Richard A. (1999). "Importance of Controlling Mobile Phase pH in Reversed Phase HPLC". Keystone Technical Bulletin.
- ↑ Schoenmakers, Peter J.; van Molle, Sylvie; Hayes, Carmel M. G.; Uunk, Louis G. M. (1991). "Effects of pH in reversed-phase liquid chromatography". Analytica Chimica Acta. 250: 1–19. doi:10.1016/0003-2670(91)85058-Z. ISSN 0003-2670.
- ↑ Dolan, John (2017-01-01). "Back to Basics: The Role of pH in Retention and Selectivity". LCGC North America. LCGC North America-01-01-2017. 35 (1): 22–28.
- ↑ "How does an acid pH affect reversed-phase chromatography separations?". www.biotage.com. Retrieved 2023-10-18.
- ↑ Kirkland, J. J.; van Straten, M. A.; Claessens, H. A. (1995). "High pH mobile phase effects on silica-based reversed-phase high-performance liquid chromatographic columns". Journal of Chromatography A. 18th International Symposium On Column Liquid Chromatography Part I. 691 (1): 3–19. doi:10.1016/0021-9673(94)00631-I. ISSN 0021-9673. S2CID 53646741.
- ↑ Dolan, John. "A Guide to HPLC and LC-MS Buffer Selection" (PDF).
- ↑ V. Agrahari; M. Bajpai; S. Nanda (2013). "Essential Concepts of Mobile Phase Selection for Reversed Phase HPLC". Research J. Pharm. And Tech. 6 (5): 459–464.
- ↑ Gilar, Martin; Fountain, Kenneth J.; Budman, Yeva; Neue, Uwe D.; Yardley, Kurt R.; Rainville, Paul D.; Russell II, Reb J.; Gebler, John C. (2002). "Ion-pair reversed-phase high-performance liquid chromatography analysis of oligonucleotides:: Retention prediction". Journal of Chromatography A. 958 (1): 167–182. doi:10.1016/S0021-9673(02)00306-0. ISSN 0021-9673. PMID 12134814.
External links
- Tables summarizing different types of reverse phases, and information on the functionalization process