.jpg.webp)
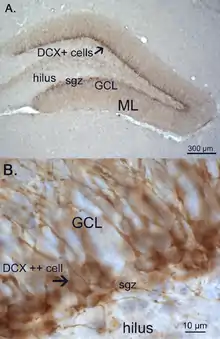
Adult neurogenesis is the process in which neurons are generated from neural stem cells in the adult. This process differs from prenatal neurogenesis.
In most mammals, new neurons are born throughout adulthood in two regions of the brain:[3]
- The subgranular zone (SGZ), part of the dentate gyrus of the hippocampus,[4][5] where neural stem cells give birth to granule cells (implicated in memory formation and learning).[6]
- The subventricular zone (SVZ) of the lateral ventricles, which can be divided into three microdomains: lateral, dorsal and medial.[7] Neural stem cells migrate to the olfactory bulb through the rostral migratory stream where they differentiate into interneurons participating in the sense of smell. In humans, however, few if any olfactory bulb neurons are generated after birth.[8][9]
More attention has been given to the neurogenesis in the dentate gyrus than in the striatum. In rodents, many of the newborn dentate gyrus neurons die shortly after they are born,[4] but a number of them become functionally integrated into the surrounding brain tissue.[10][11][12] Adult neurogenesis in rodents is reported to play a role in learning and memory, emotion, stress, depression, response to injury, and other conditions.[13]
The numbers of neurons born in the human adult hippocampus remains controversial; some studies have reported that in adult humans about 700 new neurons are added in the hippocampus every day,[14] while more recent studies show that adult hippocampal neurogenesis does not exist in humans, or, if it does, it is at undetectable levels.[15] Recent evidence shows that adult neurogenesis is basically extinct in humans.[16] The experiments advocating for the presence of adult neurogenesis have focused on how dual antigen retrieval finds that DCX antibodies are staining many cells within the adult human dentate gyrus. This finding is not as clear though as supporters of adult neurogenesis suggest; the dentate gyrus cells stained with DCX have been shown to have a mature morphology, contrasting the idea that novel neurons are being generated within the adult brain.[17] The role of new neurons in human adult brain function thus remains unclear.
Mechanism
Adult neural stem cells
Neural stem cells (NSCs) are the self-renewing, multipotent cells that generate the main phenotypes of the nervous system.
Lineage reprogramming (trans-differentiation)
Emerging evidence suggests that neural microvascular pericytes, under instruction from resident glial cells, are reprogrammed into interneurons and enrich local neuronal microcircuits.[18] This response is amplified by concomitant angiogenesis.
Model organisms of neurogenesis
Planarian
Planarian are one of the earliest model organisms used to study regeneration with Pallas as the forefather of planarian studies. Planarian are a classical invertebrate model that in recent decades have been used to examine neurogenesis. The central nervous system of a planarian is simple, though fully formed with two lobes located in the head and two ventral nerve cords. This model reproduces asexually producing a complete and fully functioning nervous system after division allowing for consistent examination of neurogenesis.
Axolotl
The axolotl is less commonly used than other vertebrates, but is still a classical model for examining regeneration and neurogenesis. Though the axolotl has made its place in biomedical research in terms of limb regeneration,[19][20] the model organism has displayed a robust ability to generate new neurons following damage .[21][22] Axolotls have contributed as a bridge organism between invertebrates and mammals, as the species has the regenerative capacity to undergo complete neurogenesis forming a wide range of neuronal populations not limited to a small niche,[23] yet the complexity and architecture is complex and analogous in many ways to human neural development.
Zebrafish
Zebrafish have long been a classical developmental model due to their transparency during organogenesis and have been utilized heavily in early development neurogenesis.[24][25]). The zebrafish displays a strong neurogenerative capacity capable of regenerating a variety of tissues and complete neuronal diversity (with the exception of astrocytes, as they have yet to be identified within the zebrafish brain) with continued neurogenesis through the life span. In recent decades the model has solidified its role in adult regeneration and neurogenesis following damage.[26][27][28][29] The zebrafish, like the axolotl, has played a key role as a bridge organism between invertebrates and mammals. The zebrafish is a rapidly developing organism that is relatively inexpensive to maintain, while providing the field ease of genetic manipulation and a complex nervous system.
Chick
Though avians have been used primarily to study early embryonic development, in recent decades the developing chick has played a critical role in the examination of neurogenesis and regeneration as the young chick is capable of neuronal-turnover at a young age, but loses the neurogenerative capacity into adulthood.[30] The loss of neuroregenerative ability over maturation has allowed investigators to further examine genetic regulators of neurogenesis.
Rodents
Rodents, mice and rats, have been the most prominent model organism since the discovery of modern neurons by Santiago Ramon y Cajal. Rodents have a very similar architecture and a complex nervous system with very little regenerative capacity similar to that found in humans. For that reason, rodents have been heavily used in pre-clinical testing. Rodents display a wide range of neural circuits responsible for complex behaviors making them ideal for studies of dendritic pruning and axonal shearing.[31] While the organism makes for a strong human analog, the model has its limitations not found in the previous models: higher cost of maintenance, lower breeding numbers, and the limited neurogenerative abilities.
To some extent, adult neurogenesis in rodents may be induced by selective disruption of Notch signalling in astrocytes:[32] this produces novel neurons which functionally integrate into the striatal circuit.[33]
Adult neurogenesis in the subventricular zone and dentate gyrus of rodents generates oxidative stress and production of reactive oxygen species that can damage both DNA and lipids.[34] The oxidative stress caused by postnatal neurogenesis may significantly contribute to the reduced learning and memory that occurs with increasing age.[34]
Octopus
A cephalopod also known as the common octopus, this organism has an intricate nervous system that demonstrates the brains capacity to produce new cells. In this case and in other taxa when compared, these organisms adapt to unpredictable environments by using newly formed brain cells.[35] This is over a short life-span (female about one year) where wild common octopuses focus most of their energy on mating and offspring care.[36][37] Findings suggest that the octopus vulgaris like other short-lived species have a complex hippocampal proliferation,[38][39] needed for spatial/navigation, and short and long-term memory.[40]
Chickadees
Black-capped chickadees are a well-known model species in the field of neuroscience for their neural mechanisms in song vocalization, plasticity, and memory. Black-capped chickadees are different from other species in the larger group of songbirds because they are characterized by food-caching behaviors. Due to this behavior, chickadees can be described through their remarkable spatial memory. Seasonal changes in hippocampal densities have been described since 1994[41] where neuronal survival peaks during the fall (October),[41] measured by thymidine (see tracking neurogenesis below) labeled cells, weeks after injection.[41] When compared to non-food caching birds such as the house sparrow, chickadees had significantly more hippocampal neuron recruitment from fall to spring.[42] The changes in hippocampal density is directly associated with increased hoarding behavior,[42] especially during the winter when better spatial memory maximizes their survival.
Over the 2 decades since the initial discovery,[41] the specific role of chickadee hippocampus in memory has gained wide attention. In an experimental setting, hippocampal lesions affect memory for locations,[43] validating previous notions for this specific role. Further, experimentally inhibiting neuronal proliferation decreases scores on spatial memory tasks,[44] supporting that new neurons hold the same role as pre-existing ones. The specific function of the hippocampus, coupled with seasonal changes in their volume, point towards their temporary advantages for spatial memory consolidation. Taken all together, adult neurogenesis in the hippocampus of black-capped chickadees suggest a selective mechanisms for neuronal survival in direct correlation with seasonal food caching behavior.
Developmentally, progenitor cells called radial glial cells are thought to mitigate newly born neurons to their destinations.[45] Radial glial cells extend processes from their soma in the avian ventricular zone to the parenchyma of the adult forebrain.[46] These New neurons have been observed as early as 3 days after thymidine administration in the HVC[47] and as early as 7 days before reaching the hippocampus.[42] Avian migration of new neurons are analogous to mammalian species,[46] providing a future direction in exploring neurogenesis in mammalian species and beyond. However, captivity has been shown to reduce hippocampal volumes when compared to wild counterparts.[48] Reduced neurogenesis in captive birds may be caused by stress, lack of exercise, diminished social interaction, and limited caching opportunities.[48]
Tracking neurogenesis
The creation of new functional neurons can be measured in several ways,[49] summarized in the following sections.
DNA labelling
Labelled DNA can trace dividing cell's lineage, and determine the location of its daughter cells. A nucleic acid analog is inserted into the genome of a neuron-generating cell (such as a glial cell or neural stem cell).[50] Thymine analogs (3H) thymidine[51] and BrdU[52] are commonly used DNA labels, and are used for radiolabelling and immunohistochemistry respectively.
Fate determination via neuronal lineage markers
DNA labeling can be used in conjunction with neuronal lineage markers to determine the fate of new functional brain cells. First, incorporated labeled nucleotides are used to detect the populations of newly divided daughter cells. Specific cell types are then determined with unique differences in their expression of proteins, which can be used as antigens in an immunoassay. For example, NeuN/Fox3 and GFAP are antigens commonly used to detect neurons, glia, and ependymal cells. Ki67 is the most commonly used antigen to detect cell proliferation. Some antigens can be used to measure specific stem cell stages. For example, stem cells requires the sox2 gene to maintain pluripotency and is used to detect enduring concentrations of stem cells in CNS tissue. The protein nestin is an intermediate filament, which is essential for the radial growth of axons, and is therefore used to detect the formation of new synapses.
Cre-Lox recombination
Some genetic tracing studies utilize cre-lox recombination to bind a promoter to a reporter gene, such as lacZ or GFP gene.[50][53] This method can be used for long term quantification of cell division and labeling, whereas the previously mentioned procedures are only useful for short-term quantification.
Viral vectors
It has recently become more common to use recombinant viruses to insert the genetic information encoding specific markers (usually protein fluorophores such as GFP) that are only expressed in cells of a certain kind. The marker gene is inserted downstream of a promoter, leading to transcription of that marker only in cells containing the transcription factor(s) that bind to that promoter. For example, a recombinant plasmid may contain the promoter for doublecortin, a protein expressed predominantly by neurons, upstream of a sequence coding for GFP, thereby making infected cells fluoresce green upon exposure to light in the blue to ultraviolet range[54] while leaving non doublecortin expressing cells unaffected, even if they contain the plasmid. Many cells will contain multiple copies of the plasmid and the fluorphore itself, allowing the fluorescent properties to be transferred along an infected cell's lineage.
By labeling a cell that gives rise to neurons, such as a neural stem cells or neural precursor cells, one can track the creation, proliferation, and even migration of newly created neurons.[55] It is important to note, however, that while the plasmid is stable for long periods of time, its protein products may have highly variable half lives and their fluorescence may decrease as well as become too diluted to be seen depending on the number of round of replication they have undergone, making this method more useful for tracking self-similar neural precursor or neural stem cells rather than neurons themselves. The insertion of genetic material via a viral vector tends to be sporadic and infrequent relative to the total number of cells in a given region of tissue, making quantification of cell division inaccurate. However, the above method can provide highly accurate data with respect to when a cell was born as well as full cellular morphologies.[56]
Methods for inhibiting neurogenesis
Many studies analyzing the role of adult neurogenesis utilize a method of inhibiting cell proliferation in specific brain regions, mimicking an inhibition of neurogenesis, to observe the effects on behavior.[13]
Pharmacological inhibition
Pharmacological inhibition is widely used in various studies, as it provides many benefits. It is generally inexpensive as compared to other methods, such as irradiation, can be used on various species, and does not require any invasive procedures or surgeries for the subjects.
However, it does pose certain challenges, as these inhibitors can't be used to inhibit proliferation in specific regions, thus leading to nonspecific effects from other systems being affected. To avoid these effects, more work must be done to determine optimal doses in order to minimize the effects on systems unrelated to neurogenesis.
A common pharmacological inhibitor for adult neurogenesis is methylazoxymethanol acetate (MAM), a chemotherapeutic agent. Other cell division inhibitors commonly used in studies are cytarabine and temozolomide.
Pharmacogenetics
Another method used to study the effects of adult neurogenesis is using pharmacogenetic models. These models provide different benefits from the pharmacological route, as it allows for more specificity by targeting specific precursors to neurogenesis and specific stem cell promoters. It also allows for temporal specificity with the interaction of certain drugs. This is beneficial in looking specifically at neurogenesis in adulthood, after normal development of other regions in the brain.
The herpes simplex virus thymidine kinase (HSV-TK) has been used in studies in conjunction with antiviral drugs to inhibit adult neurogenesis. It works by targeting stem cells using glial fibrillary acidic proteins and nestin expression. These targeted stem cells undergo cell death instead of cell proliferation when exposed to antiviral drugs.
Cre protein is also commonly used in targeting stem cells that will undergo gene changes upon treatment with tamoxifen.
Irradiation
Irradiation, the process of exposing parts of something or someone to radiation, is a method that allows for very specific inhibition of adult neurogenesis. It can be targeted to the brain to avoid affecting other systems and having nonspecific effects. It can even be used to target specific brain regions, which is important in determining how adult neurogenesis in different areas of the brain affects behavior.
Irradiation has previously been tested in adult rats, with no significant changes in cognition having been reported. However, neurogenesis in this study was stopped from progressing when the irradiation was specifically directed towards the hippocampus.[57]
However, irradiation is more expensive than the other methods and also requires large equipment with trained individuals.
Inhibition of adult neurogenesis in the hippocampus
Many studies have observed how inhibiting adult neurogenesis in other mammals, such as rats and mice, affect their behavior.[13] Inhibition of adult neurogenesis in the hippocampus has been shown to have various affects on learning and memory, conditioning, and investigative behaviors.
Impaired fear conditioning has been seen in studies involving rats with a lack of adult neurogenesis in the hippocampus.[58] Inhibition of adult neurogenesis in the hippocampus has also been linked to changes in behavior in tasks involving investigation.[59] Rats also show decreased contextualized freezing behaviors in response to contextualized fear and impairment in learning spatial locations when lacking adult neurogenesis.[60][61]
Effects on pattern separation
The changes in learning and memory seen in the studies mentioned previously are thought to be related to the role of adult neurogenesis in regulating pattern separation.[13] Pattern separation is defined as, "a process to remove redundancy from similar inputs so that events can be separated from each other and interference can be reduced, and in addition can produce a more orthogonal, sparse, and categorized set of outputs."[62]
This impairment in pattern separation could explain the impairments seen in other learning and memory tasks. A decreased ability in reducing interference could lead to greater difficulty in forming and retaining new memories.,[13] although it's hard to discriminate between effects of neurogenesis in learning and pattern separation due to limitations in the interpretation behavioral results."[63]
Studies show that rats with inhibited adult neurogenesis demonstrate difficulty in differentiating and learning contextualized fear conditioning.[13] Rats with blocked adult neurogenesis also show impaired differential freezing when they are required to differentiate between similar contexts.[64] This also affects their spatial recognition in radial arm maze tests when the arms are closer together rather than when they are further apart.[65] A meta-analysis of behavioral studies evaluating the effect of neurogenesis in different pattern separation tests has shown a consistent effect of neurogenesis ablation on performance, although there are exceptions in the literature. "[66]
Effects on behavioral inhibition
Behavioral inhibition is important in rats and other animals in halting whatever they are currently doing in order to reassess a situation in response to a threat or anything else that may require their attention.[13]
Rats with lesioned hippocampi show less behavioral inhibition when exposed to threats, such as cat odor.[67] The disruption of normal cell proliferation and development of the dentate gyrus in developing rats also impairs their freezing response, which is an example of behavior inhibition, when exposed to an unfamiliar adult male rat.[68]
This impairment in behavioral inhibition also ties into the process of learning and memory, as repressing wrong answers or behaviors requires the ability to inhibit that response.[13]
Implications
Role in learning
The functional relevance of adult neurogenesis is uncertain,[69] but there is some evidence that hippocampal adult neurogenesis is important for learning and memory.[70] Multiple mechanisms for the relationship between increased neurogenesis and improved cognition have been suggested, including computational theories to demonstrate that new neurons increase memory capacity,[71] reduce interference between memories,[72] or add information about time to memories.[73] Given that the rate of the neurogenesis does not change substantially during the adulthood, it has been proposed that unique episodic memories can be created by simply relying on the increased capacity of the young neurons of a particular age to establish stable new synapses with peers representing the unique features of an event to be memorized [74] Experiments aimed at ablating neurogenesis have proven inconclusive, but several studies have proposed neurogenic-dependence in some types of learning,[75] and others seeing no effect.[76] Studies have demonstrated that the act of learning itself is associated with increased neuronal survival.[77] However, the overall findings that adult neurogenesis is important for any kind of learning are equivocal.
Alzheimer's disease
Some studies suggest that decreased hippocampal neurogenesis can lead to development of Alzheimer's disease (AD).[78] Yet, others hypothesize that AD patients have increased neurogenesis in the CA1 region of Ammon's horn (the principal region of AD hippocampal pathology) in order to compensate for neuronal loss.[79] While the exact nature of the relationship between neurogenesis and Alzheimer's disease is unknown, insulin-like growth factor 1-stimulated neurogenesis produces major changes in hippocampal plasticity and seems to be involved in Alzheimer's pathology.[80] Allopregnanolone, a neurosteroid, aids the continued neurogenesis in the brain. Levels of allopregnanolone in the brain decline in old age and Alzheimer's disease.[81] Allopregnanolone has been shown through reversing impairment of neurogenesis to reverse the cognitive deficits in a mouse model of Alzheimer's disease.[82] Eph receptors and ephrin signaling have been shown to regulate adult neurogenesis in the hippocampus and have been studied as potential targets to treat some symptoms of AD.[83] Molecules associated with the pathology of AD, including ApoE, PS1 and APP, have also been found to impact adult neurogenesis in the hippocampus.[84]
Role in schizophrenia
Studies suggest that people with schizophrenia have a reduced hippocampus volume, which is believed to be caused by a reduction of adult neurogenesis. Correspondingly, this phenomenon might be the underlying cause of many of the symptoms of the disease. Furthermore, several research papers referred to four genes, dystrobrevin binding protein 1 (DTNBP1), neuregulin 1 (NRG1), disrupted in schizophrenia 1 (DISC1), and neuregulin 1 receptor (ERBB4), as being possibly responsible for this deficit in the normal regeneration of neurons.[85][86] Similarities between depression and schizophrenia suggest a possible biological link between the two diseases. However, further research must be done in order to clearly demonstrate this relationship.[87]
Adult neurogenesis and major depressive disorder
Research indicates that adult hippocampal neurogenesis is inversely related to major depressive disorder (MDD).[88] Neurogenesis is decreased in the hippocampus of animal models of major depressive disorder, and many treatments for the disorder, including antidepressant medication and electroconvulsive therapy, increase hippocampal neurogenesis. It has been theorized that decreased hippocampal neurogenesis in individuals with major depressive disorder may be related to the high levels of stress hormones called glucocorticoids, which are also associated with the disorder. The hippocampus instructs the hypothalamic-pituitary-adrenal axis to produce fewer glucocorticoids when glucocorticoid levels are high. A malfunctioning hippocampus, therefore, might explain the chronically high glucocorticoid levels in individuals with major depressive disorder. However, some studies have indicated that hippocampal neurogenesis is not lower in individuals with major depressive disorder and that blood glucocorticoid levels do not change when hippocampal neurogenesis changes, so the associations are still uncertain.
Stress and depression
Many now believe stress to be the most significant factor for the onset of depression. As discussed above, hippocampal cells are sensitive to stress which can lead to decreased neurogenesis. This area is being considered more frequently when examining the causes and treatments of depression. Studies have shown that removing the adrenal gland in rats caused increased neurogenesis in the dentate gyrus.[89] The adrenal gland is responsible for producing cortisol in response to a stressor, a substance that when produced in chronic amounts causes the down regulation of serotonin receptors and suppresses the birth of neurons.[90] It was shown in the same study that administration of corticosterone to normal animals suppressed neurogenesis, the opposite effect.[89] The most typical class of antidepressants administered for this disease are selective serotonin reuptake inhibitors (SSRIs)[91] and their efficacy may be explained by neurogenesis. In a normal brain, an increase in serotonin causes suppression of the corticotropin-releasing hormone (CRH) through connection to the hippocampus. It directly acts on the paraventricular nucleus to decrease CRH release and down regulate norepinephrine functioning in the locus coeruleus.[89] Because CRH is being repressed, the decrease in neurogenesis that is associated with elevated levels of it is also being reversed. This allows for the production of more brain cells, in particular at the 5-HT1a receptor in the dentate gyrus of the hippocampus which has been shown to improve symptoms of depression. It normally takes neurons approximately three to six weeks to mature,[92] which is approximately the same amount of time it takes for SSRIs to take effect. This correlation strengthens the hypothesis that SSRIs act through neurogenesis to decrease the symptoms of depression. Some neuroscientists have expressed skepticism that neurogenesis is functionally significant, given that a tiny number of nascent neurons are actually integrated into existing neural circuitry. However, a recent study used the irradiation of nascent hippocampal neurons in non-human primates (NHP) to demonstrate that neurogenesis is required for antidepressant efficacy.[93]
Adult-born neurons appear to have a role in the regulation of stress.[94][95] Studies have linked neurogenesis to the beneficial actions of specific antidepressants, suggesting a connection between decreased hippocampal neurogenesis and depression.[96][97] In a pioneer study, scientists demonstrated that the behavioral benefits of antidepressant administration in mice is reversed when neurogenesis is prevented with x-irradiation techniques.[98] In fact, newborn neurons are more excitable than older neurons due to a differential expression of GABA receptors.[99] A plausible model, therefore, is that these neurons augment the role of the hippocampus in the negative feedback mechanism of the HPA-axis (physiological stress) and perhaps in inhibiting the amygdala (the region of brain responsible for fearful responses to stimuli). Indeed, suppression of adult neurogenesis can lead to an increased HPA-axis stress response in mildly stressful situations.[94] This is consistent with numerous findings linking stress-relieving activities (learning, exposure to a new yet benign environment, and exercise) to increased levels of neurogenesis, as well as the observation that animals exposed to physiological stress (cortisol) or psychological stress (e.g. isolation) show markedly decreased levels of newborn neurons. Under chronic stress conditions, the elevation of newborn neurons by antidepressants improves the hippocampal-dependent control on the stress response; without newborn neurons, antidepressants are unable to restore the regulation of the stress response and recovery becomes impossible.[95]
Some studies have hypothesized that learning and memory are linked to depression, and that neurogenesis may promote neuroplasticity. One study proposes that mood may be regulated, at a base level, by plasticity, and thus not chemistry. Accordingly, the effects of antidepressant treatment would only be secondary to change in plasticity.[100] However another study has demonstrated an interaction between antidepressants and plasticity; the antidepressant fluoxetine has been shown to restore plasticity in the adult rat brain.[101] The results of this study imply that instead of being secondary to changes in plasticity, antidepressant therapy could promote it.
Effects of sleep reduction
One study has linked lack of sleep to a reduction in rodent hippocampal neurogenesis. The proposed mechanism for the observed decrease was increased levels of glucocorticoids. It was shown that two weeks of sleep deprivation acted as a neurogenesis-inhibitor, which was reversed after return of normal sleep and even shifted to a temporary increase in normal cell proliferation.[102] More precisely, when levels of corticosterone are elevated, sleep deprivation inhibits this process. Nonetheless, normal levels of neurogenesis after chronic sleep deprivation return after 2 weeks, with a temporary increase of neurogenesis.[103] While this is recognized, overlooked is the blood glucose demand exhibited during temporary diabetic hypoglycemic states. The American Diabetes Association amongst many documents the pseudosenilia and agitation found during temporary hypoglycemic states. Much more clinical documentation is needed to competently demonstrate the link between decreased hematologic glucose and neuronal activity and mood.
Possible use in treating Parkinson's disease
Parkinson's disease is a neurodegenerative disorder characterized by a progressive loss of dopaminergic neurons in the substantia nigra. Transplantation of fetal dopaminergic precursor cells has paved the way for the possibility of a cell replacement therapy that could ameliorate clinical symptoms in affected patients.[104] In recent years, scientists have provided evidence for the existence of neural stem cells with the potential to produce new neurons, particularly of a dopaminergic phenotype, in the adult mammalian brain.[105][106][107] Experimental depletion of dopamine in rodents decreases precursor cell proliferation in both the subependymal zone and the subgranular zone.[108] Proliferation is restored completely by a selective agonist of D2-like (D2L) receptors.[108] Neural stem cells have been identified in the neurogenic brain regions, where neurogenesis is constitutively ongoing, but also in the non-neurogenic zones, such as the midbrain and the striatum, where neurogenesis is not thought to occur under normal physiological conditions.[104] Newer research has shown that there in fact is neurogenesis in the striatum.[109] A detailed understanding of the factors governing adult neural stem cells in vivo may ultimately lead to elegant cell therapies for neurodegenerative disorders such as Parkinson's disease by mobilizing autologous endogenous neural stem cells to replace degenerated neurons.[104]
Traumatic brain injury
Traumatic brain injuries vary in their mechanism of injury, producing a blunt or penetrating trauma resulting in a primary and secondary injury with excitotoxicity and relatively wide spread neuronal death. Due to the overwhelming number of traumatic brain injuries as a result of the War on Terror, tremendous amounts of research have been placed towards a better understanding of the pathophysiology of traumatic brain injuries as well as neuroprotective interventions and possible interventions prompting restorative neurogenesis. Hormonal interventions, such as progesterone, estrogen, and allopregnanolone have been examined heavily in recent decades as possible neuroprotective agents following traumatic brain injuries to reduce the inflammation response stunt neuronal death.[110][111][112][113] In rodents, lacking the regenerative capacity for adult neurogenesis, the activation of stem cells following administration of α7 nicotinic acetylcholine receptor agonist, PNU-282987, has been identified in damaged retinas with follow-up work examining activation of neurogenesis in mammals after traumatic brain injury.[114] Currently, there is no medical intervention that has passed phase-III clinical trials for use in the human population.
Factors affecting
Changes in old age
Neurogenesis is substantially reduced in the hippocampus of aged animals, raising the possibility that it may be linked to age-related declines in hippocampal function. For example, the rate of neurogenesis in aged animals is predictive of memory.[115] However, new born cells in aged animals are functionally integrated.[116] Given that neurogenesis occurs throughout life, it might be expected that the hippocampus would steadily increase in size during adulthood, and that therefore the number of granule cells would be increased in aged animals. However, this is not the case, indicating that proliferation is balanced by cell death. Thus, it is not the addition of new neurons into the hippocampus that seems to be linked to hippocampal functions, but rather the rate of turnover of granule cells.[117]
Effects of exercise
Scientists have shown that physical activity in the form of voluntary exercise results in an increase in the number of newborn neurons in the hippocampus of mice and rats.[118][119] These and other studies have shown that learning in both species can be enhanced by physical exercise.[120] Recent research has shown that brain-derived neurotrophic factor and insulin-like growth factor 1 are key mediators of exercise-induced neurogenesis.[119][121] Exercise increases the production of BDNF, as well as the NR2B subunit of the NMDA receptor.[119] Exercise increases the uptake of IGF-1 from the bloodstream into various brain regions, including the hippocampus. In addition, IGF-1 alters c-fos expression in the hippocampus. When IGF-1 is blocked, exercise no longer induces neurogenesis.[121] Other research demonstrated that exercising mice that did not produce beta-endorphin, a mood-elevating hormone, had no change in neurogenesis. Yet, mice that did produce this hormone, along with exercise, exhibited an increase in newborn cells and their rate of survival.[122] While the association between exercise-mediated neurogenesis and enhancement of learning remains unclear, this study could have strong implications in the fields of aging and/or Alzheimer's disease.
Effects of cannabinoids
Some studies have shown that the stimulation of the cannabinoids results in the growth of new nerve cells in the hippocampus from both embryonic and adult stem cells. In 2005 a clinical study of rats at the University of Saskatchewan showed regeneration of nerve cells in the hippocampus.[123] Studies have shown that a synthetic drug resembling THC, the main psychoactive ingredient in marijuana, provides some protection against brain inflammation, which might result in better memory at an older age. This is due to receptors in the system that can also influence the production of new neurons.[124] Nonetheless, a study directed at Rutgers University demonstrated how synchronization of action potentials in the hippocampus of rats was altered after THC administration. Lack of synchronization corresponded with impaired performance in a standard test of memory.[125] Recent studies indicate that a natural cannabinoid of cannabis, cannabidiol (CBD), increases adult neurogenesis while having no effect on learning. THC however impaired learning and had no effect on neurogenesis.[126] A greater CBD to THC ratio in hair analyses of cannabis users correlates with protection against gray matter reduction in the right hippocampus.[127] CBD has also been observed to attenuate the deficits in prose recall and visuo-spatial associative memory of those currently under the influence of cannabis,[128][129] implying neuroprotective effects against heavy THC exposure. Neurogenesis might play a role in its neuroprotective effects, but further research is required.
A few studies have reported a positive association between THC and hippocampal neurogenesis.[130][131] Some of them hypotethize a biphasic effect,[130] some of them express that part of the negative effects could be attributable to neuroadaptation due to exposure at a specific period of life, and that it could be reversed.[132]
Regulation
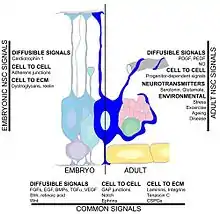
Many factors may affect the rate of hippocampal neurogenesis. Exercise and an enriched environment have been shown to promote the survival of neurons and the successful integration of newborn cells into the existing hippocampus.[133][134][135][136] Another factor is central nervous system injury since neurogenesis occurs after cerebral ischemia,[137] epileptic seizures,[138] and bacterial meningitis.[139] On the other hand, conditions such as chronic stress, viral infection and aging can result in a decreased neuronal proliferation.[140][141][142][143] Circulating factors within the blood may reduce neurogenesis. In healthy aging humans, the plasma and cerebrospinal fluid levels of certain chemokines are elevated. In a mouse model, plasma levels of these chemokines correlate with reduced neurogenesis, suggesting that neurogenesis may be modulated by certain global age-dependent systemic changes. These chemokines include CCL11, CCL2 and CCL12, which are highly localized on mouse and human chromosomes, implicating a genetic locus in aging.[70] Another study implicated the cytokine, IL-1beta, which is produced by glia. That study found that blocking IL-1 could partially prevent the severe impairment of neurogenesis caused by a viral infection.[144]
Epigenetic regulation also plays a large role in neurogenesis. DNA methylation is critical in the fate-determination of adult neural stem cells in the subventricular zone for post-natal neurogenesis through the regulation of neuronic genes such as Dlx2, Neurog2, and Sp8. Many microRNAs such as miR-124 and miR-9 have been shown to influence cortical size and layering during development.[145]
ephrin-A2 and ephrin-A3 have been showed to negatively regulate adult neurogenesis.[146]
History
Early neuroanatomists, including Santiago Ramón y Cajal, considered the nervous system fixed and incapable of regeneration. The first evidence of adult mammalian neurogenesis in the cerebral cortex was presented by Joseph Altman in 1962,[147] followed by a demonstration of adult neurogenesis in the dentate gyrus of the hippocampus in 1963.[148] In 1969, Joseph Altman discovered and named the rostral migratory stream as the source of adult generated granule cell neurons in the olfactory bulb.[149] Up until the 1980s, the scientific community ignored these findings despite use of the most direct method of demonstrating cell proliferation in the early studies, i.e. 3H-thymidine autoradiography. By that time, Shirley Bayer[150][151] (and Michael Kaplan) again showed that adult neurogenesis exists in mammals (rats), and Nottebohm showed the same phenomenon in birds[152] sparking renewed interest in the topic. Studies in the 1990s[153][154] finally put research on adult neurogenesis into a mainstream pursuit. Also in the early 1990s hippocampal neurogenesis was demonstrated in non-human primates and humans.[155][156] More recently, neurogenesis in the cerebellum of adult rabbits has also been characterized.[157] Further, some authors (particularly Elizabeth Gould) have suggested that adult neurogenesis may also occur in regions within the brain not generally associated with neurogenesis including the neocortex.[158][159][160] However, others[161] have questioned the scientific evidence of these findings, arguing that the new cells may be of glial origin. Recent research has elucidated the regulatory effect of GABA on neural stem cells. GABA's well-known inhibitory effects across the brain also affect the local circuitry that triggers a stem cell to become dormant. They found that diazepam (Valium) has a similar effect.[162]
See also
References
- ↑ Faiz M, Acarin L, Castellano B, Gonzalez B (2005). "Proliferation dynamics of germinative zone cells in the intact and excitotoxically lesioned postnatal rat brain". BMC Neuroscience. 6: 26. doi:10.1186/1471-2202-6-26. PMC 1087489. PMID 15826306.
- ↑ Oomen CA, Girardi CE, Cahyadi R, et al. (2009). Baune B (ed.). "Opposite effects of early maternal deprivation on neurogenesis in male versus female rats". PLOS ONE. 4 (1): e3675. Bibcode:2009PLoSO...4.3675O. doi:10.1371/journal.pone.0003675. PMC 2629844. PMID 19180242.
- ↑ Ernst A, Frisén J (January 2015). "Adult neurogenesis in humans- common and unique traits in mammals". PLOS Biology. 13 (1): e1002045. doi:10.1371/journal.pbio.1002045. PMC 4306487. PMID 25621867.
- 1 2 Dayer A, Ford A, Cleaver K, Yassaee M, Cameron H (2003). "Short-term and long-term survival of new neurons in the rat dentate gyrus". The Journal of Comparative Neurology. 460 (4): 563–572. doi:10.1002/cne.10675. PMID 12717714. S2CID 7153599.
- ↑ Vadodaria KC, Gage FH (2014). "SnapShot: Adult Hippocampal Neurogenesis". Cell. 156 (5): 1114–1114.e1. doi:10.1016/j.cell.2014.02.029. PMID 24581504.
- ↑ Duan X, Kang E, Liu CY, Ming G, Song H (February 2008). "Development of neural stem cell in the adult brain". Current Opinion in Neurobiology. 18 (1): 108–115. doi:10.1016/j.conb.2008.04.001. ISSN 0959-4388. PMC 2464621. PMID 18514504.
- ↑ Fiorelli R, Azim K, Fischer B, Raineteau O (2015-06-15). "Adding a spatial dimension to postnatal ventricular-subventricular zone neurogenesis". Development. 142 (12): 2109–2120. doi:10.1242/dev.119966. ISSN 1477-9129. PMID 26081572.
- ↑ Ernst A, Alkass K, Bernard S, Salehpour M, Perl S, Tisdale J, Possnert G, Druid H, Frisén J (2014). "Neurogenesis in the striatum of the adult human brain". Cell. 156 (5): 1072–83. doi:10.1016/j.cell.2014.01.044. PMID 24561062.
- ↑ Bergmann O, Liebel J, Bernard S, Alkass K, Yeung M, Steier P, Kutschera W, Johnson L, Landen M, Druid H, Spalding K, Frisen J (2012). "The age of olfactory bulb neurons in humans". Neuron. 74 (4): 634–639. doi:10.1016/j.neuron.2012.03.030. PMID 22632721.
- ↑ Toni N, Teng E, Bushong E, Aimone J, Zhao C, Consiglio A, van Praag H, Martone M, Ellisman M, Gage F (2007). "Synapse formation on neurons born in the adult hippocampus". Nature Neuroscience. 10 (6): 727–734. doi:10.1038/nn1908. PMID 17486101. S2CID 6796849.
- ↑ Vivar C, Potter M, Choi J, Lee J, Stringer T, Callawy E, Gage F, Suh H, van Praag H (2012). "Monosynaptic inputs to new neurons in the dentate gyrus". Nature Communications. 3 (1038): 1107. Bibcode:2012NatCo...3.1107V. doi:10.1038/ncomms2101. PMC 4603575. PMID 23033083.
- ↑ Toni N, Laplagne D, Zhao C, Lombardi G, Ribak C, Gage F, Schinder A (2008). "Neurons born in the adult dentate gyrus form functional synapses with target cells". Nature Neuroscience. 11 (8): 901–907. doi:10.1038/nn.2156. PMC 2572641. PMID 18622400.
- 1 2 3 4 5 6 7 8 Cameron HA, Glover LR (2015-01-03). "Adult Neurogenesis: Beyond Learning and Memory". Annual Review of Psychology. 66 (1): 53–81. doi:10.1146/annurev-psych-010814-015006. ISSN 0066-4308. PMC 5612417. PMID 25251485.
- ↑ Spalding KL, Bergmann O, Alkass K, Bernard S, Salehpour M, Huttner HB, Boström E, Westerlund I, Vial C (2013-06-06). "Dynamics of hippocampal neurogenesis in adult humans". Cell. 153 (6): 1219–1227. doi:10.1016/j.cell.2013.05.002. ISSN 1097-4172. PMC 4394608. PMID 23746839.
- ↑ Sorrells SF, Paredes MF, Cebrian-Silla A, Sandoval K, Qi D, Kelley KW, James D, Mayer S, Chang J, Auguste KI, Chang EF, Gutierrez AJ, Kriegstein AR, Mathern GW, Oldham MC, Huang EJ, Garcia-Verdugo JM, Yang Z, Alvarez-Buylla A (15 March 2018). "Human hippocampal neurogenesis drops sharply in children to undetectable levels in adults". Nature. 555 (7696): 377–381. Bibcode:2018Natur.555..377S. doi:10.1038/nature25975. PMC 6179355. PMID 29513649.
- ↑ Duque A, Arellano JI, Rakic P (January 2022). "An assessment of the existence of adult neurogenesis in humans and value of its rodent models for neuropsychiatric diseases". Molecular Psychiatry. 27 (1): 377–382. doi:10.1038/s41380-021-01314-8. ISSN 1476-5578. PMC 8967762. PMID 34667259.
- ↑ Paredes M, Sorrells S, Zhang Z, Pastor-Alonso O (March 24, 2021). "Positive Controls in Adults and Children Support That Very Few, If Any, New Neurons Are Born in the Adult Human Hippocampus". The Journal of Neuroscience: 2554–2565.
- ↑ Farahani RM, Rezaei-Lotfi S, Simonian M, Xaymardan M, Hunter N (2019). "Neural microvascular pericytes contribute to human adult neurogenesis". Journal of Comparative Neurology. 527 (4): 780–796. doi:10.1002/cne.24565. ISSN 1096-9861. PMID 30471080. S2CID 53711787.
- ↑ Carlson BM (December 1975). "The effects of rotation and positional change of stump tissues upon morphogenesis of the regenerating axolotl limb". Developmental Biology. 47 (2): 269–291. doi:10.1016/0012-1606(75)90282-1. hdl:1874/15361. ISSN 0012-1606. PMID 1204936.
- ↑ Kragl M, Knapp D, Nacu E, Khattak S, Maden M, Epperlein HH, Tanaka EM (2009-07-02). "Cells keep a memory of their tissue origin during axolotl limb regeneration". Nature. 460 (7251): 60–65. Bibcode:2009Natur.460...60K. doi:10.1038/nature08152. ISSN 1476-4687. PMID 19571878. S2CID 4316677.
- ↑ Maden M, Manwell LA, Ormerod BK (2013-01-17). "Proliferation zones in the axolotl brain and regeneration of the telencephalon". Neural Development. 8: 1. doi:10.1186/1749-8104-8-1. ISSN 1749-8104. PMC 3554517. PMID 23327114.
- ↑ Clarke JD, Alexander R, Holder N (1988-06-17). "Regeneration of descending axons in the spinal cord of the axolotl". Neuroscience Letters. 89 (1): 1–6. doi:10.1016/0304-3940(88)90471-5. ISSN 0304-3940. PMID 3399135. S2CID 23650500.
- ↑ Amamoto R, Huerta VG, Takahashi E, Dai G, Grant AK, Fu Z, Arlotta P (2016). "Adult axolotls can regenerate original neuronal diversity in response to brain injury". eLife. 5. doi:10.7554/eLife.13998. ISSN 2050-084X. PMC 4861602. PMID 27156560.
- ↑ Zupanc GK (2006-06-01). "Neurogenesis and neuronal regeneration in the adult fish brain". Journal of Comparative Physiology A. 192 (6): 649–670. doi:10.1007/s00359-006-0104-y. ISSN 0340-7594. PMID 16463148. S2CID 24063389.
- ↑ Schmidt R, Strähle U, Scholpp S (2013-02-21). "Neurogenesis in zebrafish – from embryo to adult". Neural Development. 8: 3. doi:10.1186/1749-8104-8-3. ISSN 1749-8104. PMC 3598338. PMID 23433260.
- ↑ Hentig JT, Byrd-Jacobs CA (2016-08-31). "Exposure to Zinc Sulfate Results in Differential Effects on Olfactory Sensory Neuron Subtypes in Adult Zebrafish". International Journal of Molecular Sciences. 17 (9): 1445. doi:10.3390/ijms17091445. ISSN 1422-0067. PMC 5037724. PMID 27589738.
- ↑ Gorsuch RA, Hyde DR (June 2014). "Regulation of Müller glial dependent neuronal regeneration in the damaged adult zebrafish retina". Experimental Eye Research. 123: 131–140. doi:10.1016/j.exer.2013.07.012. ISSN 1096-0007. PMC 3877724. PMID 23880528.
- ↑ Trimpe DM, Byrd-Jacobs CA (2016). "Patterns of olfactory bulb neurogenesis in the adult zebrafish are altered following reversible deafferentation". Neuroscience. 331: 134–147. doi:10.1016/j.neuroscience.2016.06.026. PMC 6496944. PMID 27343831.
- ↑ Kroehne V, Freudenreich D, Hans S, Kaslin J, Brand M (November 2011). "Regeneration of the adult zebrafish brain from neurogenic radial glia-type progenitors". Development. 138 (22): 4831–4841. doi:10.1242/dev.072587. ISSN 1477-9129. PMID 22007133.
- ↑ Fischer AJ (March 2005). "Neural regeneration in the chick retina". Progress in Retinal and Eye Research. 24 (2): 161–182. doi:10.1016/j.preteyeres.2004.07.003. ISSN 1350-9462. PMID 15610972. S2CID 43652371.
- ↑ Jones TA, Schallert T (1992-05-22). "Overgrowth and pruning of dendrites in adult rats recovering from neocortical damage". Brain Research. 581 (1): 156–160. doi:10.1016/0006-8993(92)90356-E. PMID 1498666. S2CID 34248397.
- ↑ Magnusson J (2014-10-10). "A latent neurogenic program in astrocytes regulated by Notch signaling in the mouse". Science. 346 (6206): 237–241. Bibcode:2014Sci...346..237M. doi:10.1126/science.346.6206.237. PMID 25301628. S2CID 14534396.
- ↑ Dorst M (2021-08-17). "Astrocyte-derived neurons provide excitatory input to the adult striatal circuitry". Proceedings of the National Academy of Sciences. 118 (33). Bibcode:2021PNAS..11804119D. doi:10.1073/pnas.2104119118. PMC 8379996. PMID 34389674.
- 1 2 Walton NM, Shin R, Tajinda K, Heusner CL, Kogan JH, Miyake S, Chen Q, Tamura K, Matsumoto M. Adult neurogenesis transiently generates oxidative stress. PLoS One. 2012;7(4):e35264. doi:10.1371/journal.pone.0035264. Epub 2012 Apr 30. PMID 22558133; PMCID: PMC3340368
- ↑ Di Cosmo, A. D., Bertapelle, C., Porcellini, A., & Polese, G. (2018). Magnitude Assessment of Adult Neurogenesis in the Octopus vulgaris Brain Using a Flow Cytometry-Based Technique. Frontiers in Physiology, 9. doi:10.3389/fphys.2018.01050
- ↑ Di Cosmo, A., and Polese, G. (2014). "Cephalopods meet neuroecology: the role of chemoreception in Octopus vulgaris reproductive behaviour," in Neuroecology and Neuroethology in Molluscs – The Interface Between Behaviour and Environment, eds A. Di Cosmo and W. Winlow (New York, NY: NOVA Science Publisher), 117–132.
- ↑ Polese G, Bertapelle C, Di Cosmo A (January 2015). "Role of olfaction in Octopus vulgaris reproduction". Gen. Comp. Endocrinol. 210: 55–62. doi:10.1016/j.ygcen.2014.10.006. PMID 25449183.
- ↑ Amrein I, Lipp HP (February 2009). "Adult hippocampal neurogenesis of mammals: evolution and life history". Biol. Lett. 5 (1): 141–144. doi:10.1098/rsbl.2008.0511. PMC 2657751. PMID 18957357.
- ↑ Amrein I, Isler K, Lipp HP (September 2011). "Comparing adult hippocampal neurogenesis in mammalian species and orders: influence of chronological age and life history stage" (PDF). Eur. J. Neurosci. 34 (6): 978–87. doi:10.1111/j.1460-9568.2011.07804.x. PMID 21929629. S2CID 36231667.
- ↑ Hippocampus
- 1 2 3 4 Barnea A, Nottebohm F (1994-11-08). "Seasonal recruitment of hippocampal neurons in adult free-ranging black-capped chickadees". Proceedings of the National Academy of Sciences. 91 (23): 11217–11221. Bibcode:1994PNAS...9111217B. doi:10.1073/pnas.91.23.11217. ISSN 0027-8424. PMC 45198. PMID 7972037.
- 1 2 3 Hoshooley JS, Sherry DF (March 2007). "Greater hippocampal neuronal recruitment in food-storing than in non-food-storing birds". Developmental Neurobiology. 67 (4): 406–414. doi:10.1002/dneu.20316. ISSN 1932-8451. PMID 17443797. S2CID 15930160.
- ↑ Hampton RR, Shettleworth SJ (1996). "Hippocampal lesions impair memory for location but not color in passerine birds". Behavioral Neuroscience. 110 (4): 831–835. doi:10.1037/0735-7044.110.4.831. ISSN 1939-0084. PMID 8864273.
- ↑ Hall ZJ, Delaney S, Sherry DF (2014-04-28). "Inhibition of cell proliferation in black-capped chickadees suggests a role for neurogenesis in spatial learning". Developmental Neurobiology. 74 (10): 1002–1010. doi:10.1002/dneu.22180. ISSN 1932-8451. PMID 24723376. S2CID 17537082.
- ↑ Doetsch F (2003-10-28). "The glial identity of neural stem cells". Nature Neuroscience. 6 (11): 1127–1134. doi:10.1038/nn1144. ISSN 1097-6256. PMID 14583753. S2CID 16088822.
- 1 2 Sherry DF, Hoshooley JS (2010-03-27). "Seasonal hippocampal plasticity in food-storing birds". Philosophical Transactions of the Royal Society B: Biological Sciences. 365 (1542): 933–943. doi:10.1098/rstb.2009.0220. ISSN 0962-8436. PMC 2830249. PMID 20156817.
- ↑ Kirn JR, Fishman Y, Sasportas K, Alvarez-Buylla A, Nottebohm F (1999-08-30). <487::aid-cne10>3.0.co;2-m "Fate of new neurons in adult canary high vocal center during the first 30 days after their formation". The Journal of Comparative Neurology. 411 (3): 487–494. doi:10.1002/(sici)1096-9861(19990830)411:3<487::aid-cne10>3.0.co;2-m. ISSN 0021-9967. PMID 10413781. S2CID 24242592.
- 1 2 Tarr BA, Rabinowitz JS, Imtiaz MA, DeVoogd TJ (December 2009). "Captivity reduces hippocampal volume but not survival of new cells in a food-storing bird". Developmental Neurobiology. 69 (14): 972–981. doi:10.1002/dneu.20736. ISSN 1932-8451. PMC 4597778. PMID 19813245.
- ↑ Aimone J. B., Li Y., Lee S. W., Clemenson G. D., Deng W., Gage F. H. (2014). "Regulation and Function of Adult Neurogenesis: From Genes to Cognition". Physiological Reviews. 94 (4): 991–1026. doi:10.1152/physrev.00004.2014. PMC 4280160. PMID 25287858.
- 1 2 Ming Guo-Li, Song Hongjun (2011). "Adult Neurogenesis in the Mammalian Brain: Significant Answers and Significant Questions". Neuron. 70 (4): 687–702. doi:10.1016/j.neuron.2011.05.001. PMC 3106107. PMID 21609825.
- ↑ Altman J (1962). "Are new neurons formed in the brains of adult mammals?". Science. 135 (3509): 1127–1128. Bibcode:1962Sci...135.1127A. doi:10.1126/science.135.3509.1127. PMID 13860748. S2CID 1606140.
- ↑ Kuhn HG, Cooper-Kuhn CM (2007). "Bromodeoxyuridine and the detection of neurogenesis". Curr Pharmaceutical Biotechnol. 8 (3): 127–131. doi:10.2174/138920107780906531. PMID 17584085.
- ↑ Imayoshi I, Sakamoto M, Ohtsuka T, Takao K, Miyakawa T, Yamaguchi M, Mori K, Ikeda T, Itohara S, Kageyama R (2008). "Roles of continuous neurogenesis in the structural and functional integrity of the adult forebrain". Nat Neurosci. 11 (10): 1153–1161. doi:10.1038/nn.2185. PMID 18758458. S2CID 11627647.
- ↑ Prendergast FG, Mann KG (1978-08-22). "Chemical and physical properties of aequorin and the green fluorescent protein isolated from Aequorea forskalea". Biochemistry. 17 (17): 3448–3453. doi:10.1021/bi00610a004. ISSN 0006-2960. PMID 28749.
- ↑ Gonçalves JT, Schafer ST, Gage FH (2016). "Adult Neurogenesis in the Hippocampus: From Stem Cells to Behavior". Cell. 167 (4): 897–914. doi:10.1016/j.cell.2016.10.021. PMID 27814520.
- ↑ Zhao C, Teng EM, Summers RG Jr, Ming GL, Gage FH (2006). "Distinct morphological stages of dentate granule neuron maturation in the adult mouse hippocampus". J Neurosci. 26 (1): 3–11. doi:10.1523/jneurosci.3648-05.2006. PMC 6674324. PMID 16399667.
- ↑ Lensu S, Mäkinen E, Pekkala S, Waselius T, Penttonen M, Nokia M, Kettunen H, Virtanen A, Tiirola M (March 2021). "Irradiation of the head reduces adult hippocampal neurogenesis and impairs spatial memory, but leaves overall health intact in rats". European Journal of Neuroscience. 53 (6): 1885–1904. doi:10.1111/ejn.15102. PMID 33382141. S2CID 229930018 – via EBSCO.
- ↑ Shors TJ, Miesegaes G, Beylin A, Zhao M, Rydel T, Gould E (2001). "Neurogenesis in the adult is involved in the formation of trace memories". Nature. 410 (6826): 372–376. Bibcode:2001Natur.410..372S. doi:10.1038/35066584. PMID 11268214. S2CID 4430850.
- ↑ Denny CA, Burghardt NS, Schachter DM, Hen R, Drew MR (2012-05-01). "4- to 6-week-old adult-born hippocampal neurons influence novelty-evoked exploration and contextual fear conditioning". Hippocampus. 22 (5): 1188–1201. doi:10.1002/hipo.20964. ISSN 1098-1063. PMC 3193906. PMID 21739523.
- ↑ Plack CJ, Oxenham AJ, Drga V (2006). "The Journal of Neurosci". The Journal of Neuroscience. 26 (34): 8767–8773. doi:10.1523/JNEUROSCI.1134-06.2006. PMC 1808348. PMID 16928865.
- ↑ Drew MR, Denny CA, Hen R (2010). "Arrest of adult hippocampal neurogenesis in mice impairs single- but not multiple-trial contextual fear conditioning". Behavioral Neuroscience. 124 (4): 446–454. doi:10.1037/a0020081. PMC 2925248. PMID 20695644.
- ↑ Kesner RP (2013-10-01). "An analysis of the dentate gyrus function". Behavioural Brain Research. SI:Medial Temporal Lobe Memory Networks. 254: 1–7. doi:10.1016/j.bbr.2013.01.012. PMID 23348108. S2CID 5486456.
- ↑ França TF, Bitencourt AM, Maximilla NR, Barros DM, Monserrat JM (2017-07-20). "Hippocampal neurogenesis and pattern separation: a meta-analysis of behavioral data". Hippocampus. 9. 27 (9): 937–950. doi:10.1002/hipo.22746. PMID 28597491. S2CID 205914396.
- ↑ Sahay A, Scobie KN, Hill AS, O'Carroll CM, Kheirbek MA, Burghardt NS, Fenton AA, Dranovsky A, Hen R (2011). "Increasing adult hippocampal neurogenesis is sufficient to improve pattern separation". Nature. 472 (7344): 466–470. Bibcode:2011Natur.472..466S. doi:10.1038/nature09817. PMC 3084370. PMID 21460835.
- ↑ Clelland CD, Choi M, Romberg C, Clemenson GD, Fragniere A, Tyers P, Jessberger S, Saksida LM, Barker RA (2009-07-10). "A functional role for adult hippocampal neurogenesis in spatial pattern separation". Science. 325 (5937): 210–213. Bibcode:2009Sci...325..210C. doi:10.1126/science.1173215. ISSN 1095-9203. PMC 2997634. PMID 19590004.
- ↑ França TF, Bitencourt AM, Maximilla NR, Barros DM, Monserrat JM (2017-07-20). "Hippocampal neurogenesis and pattern separation: a meta-analysis of behavioral data". Hippocampus. 9. 27 (9): 937–950. doi:10.1002/hipo.22746. PMID 28597491. S2CID 205914396.
- ↑ Kheirbek MA, Drew LJ, Burghardt NS, Costantini DO, Tannenholz L, Ahmari SE, Zeng H, Fenton AA, Hen R (2013-03-06). "Differential control of learning and anxiety along the dorsoventral axis of the dentate gyrus". Neuron. 77 (5): 955–968. doi:10.1016/j.neuron.2012.12.038. ISSN 1097-4199. PMC 3595120. PMID 23473324.
- ↑ Gould E, Cameron HA (1997). "Early NMDA receptor blockade impairs defensive behavior and increases cell proliferation in the dentate gyrus of developing rats". Behav. Neurosci. 111 (1): 49–56. doi:10.1037/0735-7044.111.1.49. PMID 9109623.
- ↑ Kempermann G, Wiskott L, Gage FH (April 2004). "Functional significance of adult neurogenesis". Current Opinion in Neurobiology. 14 (2): 186–91. doi:10.1016/j.conb.2004.03.001. PMID 15082323. S2CID 6241827.
- 1 2 G Neves G, S.F. Cooke, T.V. Bliss (2008). "Synaptic plasticity, memory and the hippocampus: A neural network approach to causality". Nature Reviews Neuroscience. 9 (1): 65–75. doi:10.1038/nrn2303. PMID 18094707. S2CID 33104731.
- ↑ Becker S (2005). "A computational principle for hippocampal learning and neurogenesis". Hippocampus. 15 (6): 722–38. doi:10.1002/hipo.20095. PMID 15986407. S2CID 1316469.
- ↑ Wiskott L, Rasch MJ, Kempermann G (2006). "A functional hypothesis for adult hippocampal neurogenesis: avoidance of catastrophic interference in the dentate gyrus". Hippocampus. 16 (3): 329–43. CiteSeerX 10.1.1.408.8944. doi:10.1002/hipo.20167. PMID 16435309. S2CID 788860.
- ↑ Aimone JB, Wiles J, Gage FH (June 2006). "Potential role for adult neurogenesis in the encoding of time in new memories". Nat. Neurosci. 9 (6): 723–7. doi:10.1038/nn1707. PMID 16732202. S2CID 10586519.
- ↑ Kovács KA (September 2020). "Episodic Memories: How do the Hippocampus and the Entorhinal Ring Attractors Cooperate to Create Them?". Frontiers in Systems Neuroscience. 14: 68. doi:10.3389/fnsys.2020.559186. PMC 7511719. PMID 33013334.
- ↑ Shors TJ, Townsend DA, Zhao M, Kozorovitskiy Y, Gould E (2002). "Neurogenesis may relate to some but not all types of hippocampal-dependent learning". Hippocampus. 12 (5): 578–84. doi:10.1002/hipo.10103. PMC 3289536. PMID 12440573.
- ↑ Meshi D, Drew MR, Saxe M, et al. (June 2006). "Hippocampal neurogenesis is not required for behavioral effects of environmental enrichment". Nat. Neurosci. 9 (6): 729–31. doi:10.1038/nn1696. PMID 16648847. S2CID 11043203.
- ↑ Gould E, Beylin A, Tanapat P, Reeves A, Shors TJ (1999). "Learning enhances adult neurogenesis in the hippocampal formation". Nature Neuroscience. 2 (3): 260–265. doi:10.1038/6365. PMID 10195219. S2CID 12112156.
- ↑ Donovan MH, Yazdani U, Norris RD, Games D, German DC, Eisch AJ (2006). "Decreased adult hippocampal neurogenesis in the PDAPP mouse model of Alzheimer's disease". The Journal of Comparative Neurology. 495 (1): 70–83. doi:10.1002/cne.20840. PMID 16432899. S2CID 20559097.
- ↑ Jin K, Peel AL, Mao XO, Xie L, Cottrell BA, Henshall DC, Greenberg DA (2004). "Increased hippocampal neurogenesis in Alzheimer's disease". Proceedings of the National Academy of Sciences. 101 (1): 343–7. Bibcode:2004PNAS..101..343J. doi:10.1073/pnas.2634794100. PMC 314187. PMID 14660786.
- ↑ Foster PP, Rosenblatt KP, Kuljiš RO (2011). "Exercise-induced cognitive plasticity, implications for mild cognitive impairment and Alzheimer's disease". Frontiers in Neurology. 2: 28. doi:10.3389/fneur.2011.00028. PMC 3092070. PMID 21602910.
- ↑ Marx CE, Trost WT, Shampine LJ, et al. (December 2006). "The neurosteroid allopregnanolone is reduced in prefrontal cortex in Alzheimer's disease". Biol. Psychiatry. 60 (12): 1287–94. doi:10.1016/j.biopsych.2006.06.017. PMID 16997284. S2CID 27813000.
- ↑ Wang JM, Singh C, Liu L, Irwin RW, Chen S, Chung EJ, Thompson RF, Brinton RD (2010). "Allopregnanolone reverses neuron and cognitive deficits in a mouse model of Alzheimer's disease". Proc. Natl. Acad. Sci. U.S.A. 107 (14): 6498–6503. Bibcode:2010PNAS..107.6498W. doi:10.1073/pnas.1001422107. PMC 2851948. PMID 20231471.
- ↑ Cissé M, Checler F (2014). "Eph receptors: New players in Alzheimer's disease pathogenesis". Neurobiology of Disease. 73C: 137–149. doi:10.1016/j.nbd.2014.08.028. PMID 25193466. S2CID 28922292.
- ↑ Mu Y, Gage FH (2011). "Adult hippocampal neurogenesis and its role in Alzheimer's disease". Molecular Neurodegeneration. 6: 85. doi:10.1186/1750-1326-6-85. PMC 3261815. PMID 22192775.
- ↑ LeStrat Y (May 2009). "The role of genes involved in neuroplasticity and neurogenesis in the observation of a gene-environment interaction (GxE) in schizophrenia". Current Molecular Medicine. 9 (4): 506–18. doi:10.2174/156652409788167104. PMID 19519407.
- ↑ Schreiber R, Newman-Tancredi A (April 2014). "Improving cognition in schizophrenia with antipsychotics that elicit neurogenesis through 5-HT1A receptor activation". Neurobiology of Learning and Memory. 110: 72–80. doi:10.1016/j.nlm.2013.12.015. PMID 24423786. S2CID 28027825.
- ↑ Reif A, Schmitt A, Fritzen S, Lesch K (27 Apr 2007). "Neurogenesis and schizophrenia: dividing neurons in a divided mind?". European Archives of Psychiatry and Clinical Neuroscience. 257 (5): 290–299. doi:10.1007/s00406-007-0733-3. PMID 17468935. S2CID 28750292.
- ↑ Numakawa T, Odaka H, Adachi N (2017). "Impact of glucocorticoid on neurogenesis". Neural Regeneration Research. 12 (7): 1028–1035. doi:10.4103/1673-5374.211174. PMC 5558474. PMID 28852377.
- 1 2 3 Jacobs, B. L., H. van Praag, F. H. Gage (2000). "Depression and the Birth and Death of Brain Cells". American Scientist. 88 (4): 340. Bibcode:2000AmSci..88..340J. doi:10.1511/2000.4.340.
- ↑ Kandel, E. R., J. H. Schwartz, T. M. Jessell (2012-10-26). Principles of Neural Science (fifth ed.). McGraw Hill Professional. ISBN 978-0071390118.
{{cite book}}
: CS1 maint: overridden setting (link) - ↑ National Institute of Mental Health (2010). "Mental Health Medications" (PDF).
- ↑ Davies-Sala, M. G., Espósito, M. S., Piatti, V. C., Mongiat, L. A., Trinchero M. F., Schinder A. F. (25 May 2011). "The Timing for Neuronal Maturation in the Adult Hippocampus Is Modulated by Local Network Activity". The Journal of Neuroscience. 31 (21): 7715–28. doi:10.1523/JNEUROSCI.1380-11.2011. PMC 3701257. PMID 21613484.
{{cite journal}}
: CS1 maint: overridden setting (link) - ↑ Perera TD, Dwork AJ, Keegan KA, Thirumangalakudi L, Lipira CM, Joyce N, Lange C, Higley JD, Rosoklija G, Hen R, Sackeim HA, Coplan JD (2011). "Necessity of hippocampal neurogenesis for the therapeutic action of antidepressants in adult nonhuman primates". PLOS ONE. 6 (4): e17600. Bibcode:2011PLoSO...617600P. doi:10.1371/journal.pone.0017600. PMC 3078107. PMID 21525974.
- 1 2 Schloesser RJ, Manji HK, Martinowich K (April 2009). "Suppression of adult neurogenesis leads to an increased hypothalamo-pituitary-adrenal axis response". NeuroReport. 20 (6): 553–7. doi:10.1097/WNR.0b013e3283293e59. PMC 2693911. PMID 19322118.
- 1 2 Surget A, Tanti A, Leonardo ED, et al. (December 2011). "Antidepressants recruit new neurons to improve stress response regulation". Molecular Psychiatry. 16 (12): 1177–88. doi:10.1038/mp.2011.48. PMC 3223314. PMID 21537331.
- ↑ Malberg JE, Eisch AJ, Nestler EJ, Duman RS (December 2000). "Chronic antidepressant treatment increases neurogenesis in adult rat hippocampus". J. Neurosci. 20 (24): 9104–10. doi:10.1523/JNEUROSCI.20-24-09104.2000. PMC 6773038. PMID 11124987.
- ↑ Manev H, Uz T, Smalheiser NR, Manev R (January 2001). "Antidepressants alter cell proliferation in the adult brain in vivo and in neural cultures in vitro". Eur J Pharmacol. 411 (1–2): 67–70. doi:10.1016/S0014-2999(00)00904-3. PMID 11137860.
- ↑ Santarelli L, Saxe M, Gross C, et al. (August 2003). "Requirement of hippocampal neurogenesis for the behavioral effects of antidepressants". Science. 301 (5634): 805–9. Bibcode:2003Sci...301..805S. doi:10.1126/science.1083328. PMID 12907793. S2CID 9699898.
- ↑ Bradley J (2015). Addiction: From Suffering to Solution. Las Vegas, NV: Breaux Press International. p. 173. ISBN 978-0-9854418-0-7.
- ↑ Castrén E (March 2005). "Is mood chemistry?". Nature Reviews Neuroscience. 6 (3): 241–6. doi:10.1038/nrn1629. PMID 15738959. S2CID 34523310.
- ↑ Vetencourt JF, Sale A, Viegi A, Baroncelli L, De Pasquale R, f o'Leary O, Castren E, Maffei L (2008). "The Antidepressant Fluoxetine Restores Plasticity in the Adult Visual Cortex". Science. 320 (5874): 385–8. Bibcode:2008Sci...320..385M. doi:10.1126/science.1150516. PMID 18420937. S2CID 39565465.
- ↑ Mirescu C, Peters JD, Noiman L, Gould E (December 2006). "Sleep deprivation inhibits adult neurogenesis in the hippocampus by elevating glucocorticoids". Proc. Natl. Acad. Sci. U.S.A. 103 (50): 19170–5. Bibcode:2006PNAS..10319170M. doi:10.1073/pnas.0608644103. PMC 1748194. PMID 17135354.
- ↑ Mirescu C., Peters J. D., Noiman L., Gould E. (2006). "Sleep deprivation inhibits adult neurogenesis in the hippocampus by elevating glucocorticoids". Proceedings of the National Academy of Sciences. 103 (50): 19170–19175. Bibcode:2006PNAS..10319170M. doi:10.1073/pnas.0608644103. PMC 1748194. PMID 17135354.
- 1 2 3 Arias-Carrión O, Freundlieb N, Oertel WH, Höglinger GU (October 2007). "Adult neurogenesis and Parkinson's disease". CNS Neurol Disord Drug Targets. 6 (5): 326–35. doi:10.2174/187152707783220875. PMID 18045161. Archived from the original on 2013-04-14.
- ↑ Fallon J, Reid S, Kinyamu R, et al. (December 2000). "In vivo induction of massive proliferation, directed migration, and differentiation of neural cells in the adult mammalian brain". Proc. Natl. Acad. Sci. U.S.A. 97 (26): 14686–91. Bibcode:2000PNAS...9714686F. doi:10.1073/pnas.97.26.14686. PMC 18979. PMID 11121069.
- ↑ Arias-Carrión O, Verdugo-Díaz L, Feria-Velasco A, et al. (October 2004). "Neurogenesis in the subventricular zone following transcranial magnetic field stimulation and nigrostriatal lesions". J Neurosci Res. 78 (1): 16–28. doi:10.1002/jnr.20235. PMID 15372495. S2CID 6349942.
- ↑ Arias-Carrión O, Hernández-López S, Ibañez-Sandoval O, Bargas J, Hernández-Cruz A, Drucker-Colín R (November 2006). "Neuronal precursors within the adult rat subventricular zone differentiate into dopaminergic neurons after substantia nigra lesion and chromaffin cell transplant". J Neurosci Res. 84 (7): 1425–37. doi:10.1002/jnr.21068. PMID 17006899. S2CID 21995251.
- 1 2 Höglinger GU, Rizk P, Muriel MP, et al. (July 2004). "Dopamine depletion impairs precursor cell proliferation in Parkinson disease". Nat. Neurosci. 7 (7): 726–35. doi:10.1038/nn1265. PMID 15195095. S2CID 952173.
- ↑ Neurogenesis in the Striatum of the Adult Human Brain
- ↑ Guo Q, Sayeed I, Baronne LM, Hoffman SW, Guennoun R, Stein DG (April 2006). "Progesterone administration modulates AQP4 expression and edema after traumatic brain injury in male rats". Experimental Neurology. 198 (2): 469–478. doi:10.1016/j.expneurol.2005.12.013. ISSN 0014-4886. PMID 16445913. S2CID 26143265.
- ↑ Petrone AB, Gatson JW, Simpkins JW, Reed MN (2014-05-25). "Non-feminizing estrogens: a novel neuroprotective therapy". Molecular and Cellular Endocrinology. 389 (1–2): 40–47. doi:10.1016/j.mce.2013.12.017. ISSN 1872-8057. PMC 4040321. PMID 24424441.
- ↑ Han S, Zhao B, Pan X, Song Z, Liu J, Gong Y, Wang M (2015-12-03). "Estrogen receptor variant ER-α36 is involved in estrogen neuroprotection against oxidative toxicity". Neuroscience. 310: 224–241. doi:10.1016/j.neuroscience.2015.09.024. ISSN 1873-7544. PMID 26383254. S2CID 44289364.
- ↑ Singh S, Hota D, Prakash A, Khanduja KL, Arora SK, Chakrabarti A (January 2010). "Allopregnanolone, the active metabolite of progesterone protects against neuronal damage in picrotoxin-induced seizure model in mice". Pharmacology Biochemistry and Behavior. 94 (3): 416–422. doi:10.1016/j.pbb.2009.10.003. ISSN 1873-5177. PMID 19840816. S2CID 45591868.
- ↑ Webster MK, Cooley-Themm CA, Barnett JD, Bach HB, Vainner JM, Webster SE, Linn CL (2017-03-27). "Evidence of BrdU-positive retinal neurons after application of an Alpha7 nicotinic acetylcholine receptor agonist". Neuroscience. 346: 437–446. doi:10.1016/j.neuroscience.2017.01.029. ISSN 1873-7544. PMC 5341387. PMID 28147247.
- ↑ Drapeau, E., Mayo, W., Aurousseau, C., Moal, M.L., Piazza, P., Abrous, D.N. (2003). "Spatial memory performances of aged rats in the water maze predict levels of hippocampal neurogenesis". PNAS. 100 (24): 14385–14390. Bibcode:2003PNAS..10014385D. doi:10.1073/pnas.2334169100. PMC 283601. PMID 14614143.
{{cite journal}}
: CS1 maint: overridden setting (link) - ↑ Marrone, D.F., Ramirez-Amaya, V., Barnes, C.A. (2012). "Neurons generated in senescence maintain capacity for functional integration". Hippocampus. 22 (5): 1134–1142. doi:10.1002/hipo.20959. PMC 3367380. PMID 21695743.
{{cite journal}}
: CS1 maint: overridden setting (link) - ↑ von Bohlen und Halbach O (2010). "Involvement of BDNF in age-dependent alterations in the hippocampus". Front Aging Neurosci. 2. doi:10.3389/fnagi.2010.00036. PMC 2952461. PMID 20941325.
- ↑ Praag H, Christie BR, Sejnowski TJ, Gage FH (1999). "Running enhances neurogenesis, learning, and long-term potentiation in mice". Proc Natl Acad Sci U S A. 96 (23): 13427–31. Bibcode:1999PNAS...9613427V. doi:10.1073/pnas.96.23.13427. PMC 23964. PMID 10557337.
- 1 2 3 Farmer J, Zhao X, van Praag H, Wodtke K, Gage FH, Christie BR (2004). "Effects of voluntary exercise on synaptic plasticity and gene expression in the dentate gyrus of adult male Sprague-Dawley rats in vivo". Neuroscience. 124 (1): 71–9. doi:10.1016/j.neuroscience.2003.09.029. PMID 14960340. S2CID 2718669.
- ↑ van Praag H, Christie BR, Sejnowski TJ, Gage FH (Nov 1999). "Running enhances neurogenesis, learning, and long-term potentiation in mice". Proc Natl Acad Sci U S A. 96 (23): 13427–31. Bibcode:1999PNAS...9613427V. doi:10.1073/pnas.96.23.13427. PMC 23964. PMID 10557337.
- 1 2 Carro E, Trejo JL, Busiguina S, Torres-Aleman I (2001). "Circulating insulin-like growth factor I mediates the protective effects of physical exercise against brain insults of different etiology and anatomy". The Journal of Neuroscience. 21 (15): 5678–84. doi:10.1523/JNEUROSCI.21-15-05678.2001. PMC 6762673. PMID 11466439.
- ↑ "Adult neurogenesis". Brain Briefings. Society for Neuroscience. June 2007. Retrieved 2011-11-26.
- ↑ Wen Jiang, Yun Zhang, Lan Xiao, Jamie Van Cleemput, Shao-Ping Ji, Guang Bai, Xia Zhang (2005-11-01). "Cannabinoids promote embryonic and adult hippocampus neurogenesis and produce anxiolytic- and antidepressant-like effects". Journal of Clinical Investigation. 115 (11): 3104–16. doi:10.1172/JCI25509. PMC 1253627. PMID 16224541.
- ↑ 11-19-2008 Ohio State study: Scientists are high on idea that marijuana reduces memory impairment. OSU.edu
- ↑ Nov 29, 2006. Study: Marijuana may affect neuron firing. United Press International
- ↑ Wolf SA, Bick-Sander A, Fabel K, Leal-Galicia P, Tauber S, Ramirez-Rodriguez G, Müller A, Melnik A, Waltinger TP, Ullrich O, Kempermann G (2010). "Cannabinoid receptor CB1 mediates baseline and activity-induced survival of new neurons in adult hippocampal neurogenesis". Cell Communication and Signaling. 8 (1): 12. doi:10.1186/1478-811X-8-12. PMC 2898685. PMID 20565726.
- ↑ Demirakca T, Sartorius A, Ende G, Meyer N, Welzel H, Skopp G, Mann K, Hermann D (2010). "Diminished gray matter in the hippocampus of cannabis users: Possible protective effects of cannabidiol". Drug and Alcohol Dependence. 114 (2–3): 242–245. doi:10.1016/j.drugalcdep.2010.09.020. PMID 21050680.
- ↑ Wright MJ, Vandewater SA, Taffe MA (2013). "Cannabidiol attenuates deficits of visuospatial associative memory induced by Δ9tetrahydrocannabinol". British Journal of Pharmacology. 170 (7): 1365–1373. doi:10.1111/bph.12199. PMC 3838683. PMID 23550724.
- ↑ Morgan CJ, Schafer G, Freeman TP, Curran HV (2010). "Impact of cannabidiol on the acute memory and psychotomimetic effects of smoked cannabis: Naturalistic study". The British Journal of Psychiatry. 197 (4): 285–290. doi:10.1192/bjp.bp.110.077503. PMID 20884951.
- 1 2 Calabrese EJ, Rubio-Casillas A (May 2018). "Biphasic effects of THC in memory and cognition". European Journal of Clinical Investigation. 48 (5): e12920. doi:10.1111/eci.12920. PMID 29574698.
- ↑ Suliman NA, Taib CN, Moklas MA, Basir R (21 September 2017). "Delta-9-Tetrahydrocannabinol (∆9-THC) Induce Neurogenesis and Improve Cognitive Performances of Male Sprague Dawley Rats". Neurotoxicity Research. 33 (2): 402–411. doi:10.1007/s12640-017-9806-x. PMC 5766723. PMID 28933048.
- ↑ Cuccurazzu B, Zamberletti E, Nazzaro C, Prini P, Trusel M, Grilli M, Parolaro D, Tonini R, Rubino T (November 2018). "Adult Cellular Neuroadaptations Induced by Adolescent THC Exposure in Female Rats Are Rescued by Enhancing Anandamide Signaling". International Journal of Neuropsychopharmacology. 21 (11): 1014–1024. doi:10.1093/ijnp/pyy057. PMC 6209859. PMID 29982505.
- ↑ Lazarov O, Robinson J, Tang YP, Hairston IS, Korade-Mirnics Z, Lee VM, Hersh LB, Sapolsky RM, Mirnics K, Sisodia SS (March 2005). "Environmental enrichment reduces Abeta levels and amyloid deposition in transgenic mice". Cell. 120 (5): 701–13. doi:10.1016/j.cell.2005.01.015. PMID 15766532. S2CID 16756661.
- ↑ Van Praag H, Shubert T, Zhao C, Gage F (2005). "Exercise enhances learning and hippocampal neurogenesis in aged mice". Journal of Neuroscience. 25 (38): 8680–8685. doi:10.1523/JNEUROSCI.1731-05.2005. PMC 1360197. PMID 16177036.
- ↑ Van Praag H, Kempermann G, Gage F (1999). "Running increases cell proliferation and neurogenesis in the adult mouse dentate gyrus". Nature Neuroscience. 2 (3): 266–270. doi:10.1038/6368. PMID 10195220. S2CID 7170664.
- ↑ Bjørnebekk A, Mathé AA, Brené S (September 2005). "The antidepressant effect of running is associated with increased hippocampal cell proliferation". Int J Neuropsychopharmacol. 8 (3): 357–68. doi:10.1017/S1461145705005122. PMID 15769301.
- ↑ Jin K, Wang X, Xie L, et al. (August 2006). "Evidence for stroke-induced neurogenesis in the human brain". Proc. Natl. Acad. Sci. U.S.A. 103 (35): 13198–202. Bibcode:2006PNAS..10313198J. doi:10.1073/pnas.0603512103. PMC 1559776. PMID 16924107.
- ↑ Parent JM, Elliott RC, Pleasure SJ, Barbaro NM, Lowenstein DH (2006). "Aberrant seizure-induced neurogenesis in experimental temporal lobe epilepsy". Annals of Neurology. 59 (1): 81–91. doi:10.1002/ana.20699. hdl:2027.42/49280. PMID 16261566. S2CID 9655613.
- ↑ Gerber J, Tauber SC, Armbrecht I, Schmidt H, Brück W, Nau R (2009). "Increased neuronal proliferation in human bacterial meningitis". Neurology. 73 (13): 1026–32. doi:10.1212/WNL.0b013e3181b9c892. PMID 19786694. S2CID 26108905.
- ↑ Sharma A, Valadi N, Miller AH, Pearce BD (2002). "Adult neurogenesis in the hippocampus is impaired following neonatal viral infection". Neurobiology of Disease. 11 (2): 246–56. doi:10.1006/nbdi.2002.0531. PMID 12505418. S2CID 6405590.
- ↑ Lee AL, Ogle WO, Sapolsky RM (April 2002). "Stress and depression: possible links to neuron death in the hippocampus". Bipolar Disord. 4 (2): 117–28. doi:10.1034/j.1399-5618.2002.01144.x. PMID 12071509.
- ↑ Sheline YI, Gado MH, Kraemer HC (August 2003). "Untreated depression and hippocampal volume loss". Am J Psychiatry. 160 (8): 1516–8. doi:10.1176/appi.ajp.160.8.1516. PMID 12900317.
- ↑ Jiang, W., et al. (2005). "Cannabinoids promote embryonic and adult hippocampus neurogenesis and produce anxiolytic- and antidepressant-like effects". The Journal of Clinical Investigation. 115 (11): 3104–3116. doi:10.1172/JCI25509. PMC 1253627. PMID 16224541.
- ↑ Orr AG, Sharma A, Binder NB, Miller AH, Pearce BD (2010). "Interleukin-1 Mediates Long-Term Hippocampal Dentate Granule Cell Loss Following Postnatal Viral Infection". Journal of Molecular Neuroscience. 41 (1): 89–96. doi:10.1007/s12031-009-9293-5. PMID 19774496. S2CID 2427299.
- ↑ Hu X, Wang, Y., Shen, Q. (2012). "Epigenetic control on cell fate choice in neural stem cells". Protein & Cell. 3 (4): 278–290. doi:10.1007/s13238-012-2916-6. PMC 4729703. PMID 22549586.
- ↑ Jiao JW, Feldheim DA, Chen DF (2008). "Ephrins as negative regulators of adult neurogenesis in diverse regions of the central nervous system". Proceedings of the National Academy of Sciences of the United States of America. 105 (25): 8778–8783. Bibcode:2008PNAS..105.8778J. doi:10.1073/pnas.0708861105. PMC 2438395. PMID 18562299.
- ↑ Altman J (1962). "Are new neurons formed in the brains of adult mammals?". Science. 135 (3509): 1127–1128. Bibcode:1962Sci...135.1127A. doi:10.1126/science.135.3509.1127. PMID 13860748. S2CID 1606140.
- ↑ Altman J (1963). "Autoradiographic investigation of cell proliferation in the brains of rats and cats". The Anatomical Record. 145 (4): 573–591. doi:10.1002/ar.1091450409. PMID 14012334. S2CID 5340726.
- ↑ Altman J (1969). "Autoradiographic and histological studies of postnatal neurogenesis. IV. Cell proliferation and migration in the anterior forebrain, with special reference to persisting neurogenesis in the olfactory bulb". The Journal of Comparative Neurology. 137 (4): 433–457. doi:10.1002/cne.901370404. PMID 5361244. S2CID 46728071.
- ↑ Bayer SA, Yackel JW, Puri PS (1982). "Neurons in the rat dentate gyrus granular layer substantially increase during juvenile and adult life". Science. 216 (4548): 890–892. Bibcode:1982Sci...216..890B. doi:10.1126/science.7079742. PMID 7079742.
- ↑ Bayer SA (1982). "Changes in the total number of dentate granule cells in juvenile and adult rats: a correlated volumetric and 3H-thymidine autoradiographic study". Experimental Brain Research. Experimentelle Hirnforschung. Experimentation Cerebrale. 46 (3): 315–323. doi:10.1007/bf00238626. PMID 7095040. S2CID 18663323.
- ↑ Goldman SA, Nottebohm F (April 1983). "Neuronal production, migration, and differentiation in a vocal control nucleus of the adult female canary brain". Proc. Natl. Acad. Sci. U.S.A. 80 (8): 2390–4. Bibcode:1983PNAS...80.2390G. doi:10.1073/pnas.80.8.2390. PMC 393826. PMID 6572982.
- ↑ Reynolds BA, Weiss S (Mar 1992). "Generation of neurons and astrocytes from isolated cells of the adult mammalian central nervous system". Science. 255 (5052): 1707–1710. Bibcode:1992Sci...255.1707R. doi:10.1126/science.1553558. ISSN 0036-8075. PMID 1553558.
- ↑ Gage FH, Ray J, Fisher LJ (1995). "Isolation, Characterization, and use of Stem Cells from the CNS". Annual Review of Neuroscience. 18: 159–92. doi:10.1146/annurev.ne.18.030195.001111. PMID 7605059.
- ↑ Eriksson PS, Perfilieva E, Björk-Eriksson T, et al. (November 1998). "Neurogenesis in the adult human hippocampus". Nat. Med. 4 (11): 1313–7. doi:10.1038/3305. PMID 9809557.
- ↑ Gould E, Reeves, Fallah, Tanapat, Gross, Fuchs (1999). "Hippocampal neurogenesis in adult Old World primates". Proceedings of the National Academy of Sciences of the United States of America. 96 (9): 5263–5267. Bibcode:1999PNAS...96.5263G. doi:10.1073/pnas.96.9.5263. PMC 21852. PMID 10220454.
- ↑ Ponti G, Peretto B, Bonfanti L (2008). Reh TA (ed.). "Genesis of neuronal and glial progenitors in the cerebellar cortex of peripuberal and adult rabbits". PLOS ONE. 3 (6): e2366. Bibcode:2008PLoSO...3.2366P. doi:10.1371/journal.pone.0002366. PMC 2396292. PMID 18523645.
- ↑ Gould E, Reeves AJ, Graziano MS, Gross CG (1999). "Neurogenesis in the neocortex of adult primates". Science. 286 (5439): 548–552. doi:10.1126/science.286.5439.548. PMID 10521353.
- ↑ Zhao M, Momma S, Delfani K, Carlen M, Cassidy RM, Johansson CB, Brismar H, Shupliakov O, Frisen J, Janson A (2003). "Evidence for neurogenesis in the adult mammalian substantia nigra". Proceedings of the National Academy of Sciences of the United States of America. 100 (13): 7925–7930. Bibcode:2003PNAS..100.7925Z. doi:10.1073/pnas.1131955100. PMC 164689. PMID 12792021.
- ↑ Shankle, Rafii MS, Landing BH, Fallon JH (1999). "Approximate doubling of numbers of neurons in postnatal human cerebral cortex and in 35 specific cytoarchitectural areas from birth to 72 months". Pediatric and Developmental Pathology. 2 (3): 244–259. doi:10.1007/s100249900120. PMID 10191348. S2CID 23546857.
- ↑ Rakic P (February 2002). "Adult neurogenesis in mammals: an identity crisis". J. Neurosci. 22 (3): 614–8. doi:10.1523/JNEUROSCI.22-03-00614.2002. PMC 6758501. PMID 11826088.
- ↑ Song J, Zhong C, Bonaguidi MA, Sun GJ, Hsu D, Gu Y, Meletis K, Huang ZJ, Ge S, Enikolopov G, Deisseroth K, Luscher B, Christian KM, Ming G, Song H (2012). "Neuronal circuitry mechanism regulating adult quiescent neural stem-cell fate decision". Nature. 489 (7414): 150–154. Bibcode:2012Natur.489..150S. doi:10.1038/nature11306. PMC 3438284. PMID 22842902.
- Notes
- Aimone JB, Jessberger S, and Gage FH (2007) Adult Neurogenesis. Scholarpedia, p. 8739
- Gould E, Reeves AJ, Fallah M, Tanapat P, Gross CG, Fuchs E (April 1999). "Hippocampal neurogenesis in adult Old World primates". Proc. Natl. Acad. Sci. U.S.A. 96 (9): 5263–7. Bibcode:1999PNAS...96.5263G. doi:10.1073/pnas.96.9.5263. PMC 21852. PMID 10220454.
- Gould E, Reeves AJ, Graziano MS, Gross CG (October 1999). "Neurogenesis in the neocortex of adult primates". Science. 286 (5439): 548–52. doi:10.1126/science.286.5439.548. PMID 10521353.
- Gould E, Beylin A, Tanapat P, Reeves A, Shors TJ (March 1999). "Learning enhances adult neurogenesis in the hippocampal formation". Nat. Neurosci. 2 (3): 260–5. doi:10.1038/6365. PMID 10195219. S2CID 12112156.
- Moghadam KS, Chen A, Heathcote RD (August 2003). "Establishment of a ventral cell fate in the spinal cord". Dev. Dyn. 227 (4): 552–62. doi:10.1002/dvdy.10340. PMID 12889064. S2CID 209599.
- Rakic P (January 2002). "Neurogenesis in adult primate neocortex: an evaluation of the evidence". Nature Reviews Neuroscience. 3 (1): 65–71. doi:10.1038/nrn700. PMID 11823806. S2CID 15725675.
- Rolls, E.T & Treves, A. (1998). Neural Networks and Brain Function. Oxford: OUP. ISBN 0-19-852432-3.
- Santarelli L, Saxe M, Gross C, et al. (August 2003). "Requirement of hippocampal neurogenesis for the behavioral effects of antidepressants". Science. 301 (5634): 805–9. Bibcode:2003Sci...301..805S. doi:10.1126/science.1083328. PMID 12907793. S2CID 9699898.
- Schloesser RJ, Manji HK, Martinowich K (April 2009). "Suppression of adult neurogenesis leads to an increased hypothalamo-pituitary-adrenal axis response". NeuroReport. 20 (6): 553–7. doi:10.1097/WNR.0b013e3283293e59. PMC 2693911. PMID 19322118.
- Shankle WR, Rafii MS, Landing BH, Fallon JH (1999). "Approximate doubling of the numbers of neurons in the postnatal human cortex and in 35 specific cytoarchitectonic areas from birth to 72 months". Pediatric and Developmental Pathology. 2 (3): 244–259. doi:10.1007/s100249900120. PMID 10191348. S2CID 23546857.
- Zhao M, Momma S, Delfani K, et al. (June 2003). "Evidence for neurogenesis in the adult mammalian substantia nigra". Proc. Natl. Acad. Sci. U.S.A. 100 (13): 7925–30. Bibcode:2003PNAS..100.7925Z. doi:10.1073/pnas.1131955100. PMC 164689. PMID 12792021.
External links
- Concise introduction to neurogenesis from Wellesley College
- Comprehensive website on neurogenesis from Lafayette College
- Early literature on adult neurogenesis
- Neurogenesis in adult brain - Fred H. Gage and Henriette van Praag
- "Neurogenesis and Parkinson´s disease"
- Scholarpedia Article on Adult Neurogenesis
- "Trends in Neurosciences, 10 October 2001 (Michael S. Kaplan MD, PhD)
- New York Times: Studies Find Brains Grow New Cells
- Michael Specter: Rethinking the Brain Archived 2019-06-30 at the Wayback Machine - How the songs of canaries upset a fundamental principle of science
- The Neurogenesis Experiment - Article series on adult human neurogenesis
- Seed magazine: The Reinvention of the Self - A historical background on the field of neurogenesis and implications of this research
- BBC Radio 4: The Memory Experience - Use it or Lose it
- PBS: Changing Your Mind - Grow Your Own Brain
- Lobes of Steel: Aerobic exercise appears to promote neurogenesis, New York Times, 19 August 2007.
Media related to neurogenesis at Wikimedia Commons