The causes of schizophrenia that underlie the development of schizophrenia, a psychiatric disorder, are complex and not clearly understood. A number of hypotheses including the dopamine hypothesis, and the glutamate hypothesis have been put forward in an attempt to explain the link between altered brain function and the symptoms and development of schizophrenia.[1]
Pathophysiology
The exact pathophysiology of schizophrenia remains poorly understood. The most commonly supported theories are the dopamine hypothesis and the glutamate hypothesis.[1][2][3] Other theories include the specific dysfunction of interneurons, abnormalities in the immune system, abnormalities in myelination, and oxidative stress.[4][5][6][7][8][9]
Dopamine dysfunction
The first formulations of the dopamine hypothesis of schizophrenia came from post-mortem studies finding increased numbers of D2/D3 receptors in the striatum, and elevated cerebrospinal fluid levels of dopamine metabolites. Subsequently, most antipsychotics were found to have affinity for D2 receptors. Later investigations have suggested a link between striatal dopamine synthesis and positive symptoms, as well as increased dopamine transmission in subcortical regions, and decreased transmission in cortical regions.
A meta-analysis of molecular imaging studies observed increased presynaptic indicators of dopamine function, but no difference in the availability of dopamine transporters or dopamine D2/D3 receptors. Both studies using radio labeled L-DOPA, an indicator of dopamine synthesis, and studies using amphetamine release challenges observed significant differences between those with schizophrenia and control. These findings were interpreted as increased synthesis of dopamine, and increased release of dopamine respectively. These findings were localized to the striatum, and were noted to be limited by the quality of studies used.[10] A large degree of inconsistency has been observed in D2/D3 receptor binding, although a small but nonsignificant reduction in thalamic availability has been found.[11] The inconsistent findings with respect to receptor expression has been emphasized as not precluding dysfunction in dopamine receptors, as many factors such as regional heterogeneity and medication status may lead to variable findings. When combined with findings in presynaptic dopamine function, most evidence suggests dysregulation of dopamine in schizophrenia.[12]
Exactly how dopamine dysregulation can contribute to schizophrenia symptoms remains unclear. Some studies have suggested that disruption of the auditory thalamocortical projections give rise to hallucinations,[13] while dysregulated corticostriatal circuitry and reward circuitry in the form of aberrant salience can give rise to delusions.[14] Decreased inhibitory dopamine signals in the thalamus have been hypothesized to result in reduced sensory gating, and excessive activity in excitatory inputs into the cortex.[15]
One hypothesis linking delusions in schizophrenia to dopamine suggests that unstable representation of expectations in prefrontal neurons occurs in psychotic states due to insufficient D1 and NMDA receptor stimulation. This, when combined with hyperactivity of expectations to modification by salient stimuli is thought to lead to improper formation of beliefs.[16]
Glutamate dysfunction
Beside the dopamine hypothesis, interest has also focused on the neurotransmitter glutamate and the reduced function of the NMDA glutamate receptor in the pathophysiology of schizophrenia. This has largely been suggested by lower levels of glutamate receptors found in postmortem brains of people previously diagnosed with schizophrenia[17] and the discovery that glutamate blocking drugs such as phencyclidine and ketamine can mimic the symptoms and cognitive problems associated with the condition.[18]
The fact that reduced glutamate function is linked to poor performance on tests requiring frontal lobe and hippocampal function and that glutamate can affect dopamine function, all of which have been implicated in schizophrenia, have suggested an important mediating (and possibly causal) role of glutamate pathways in schizophrenia.[19] Positive symptoms fail however to respond to glutamatergic medication.[20]
Reduced mRNA and protein expression of several NMDA receptor subunits has also been reported in postmortem brains from people with schizophrenia.[21] In particular, the expression of mRNA for the NR1 receptor subunit, as well as the protein itself is reduced in the prefrontal cortex in post-mortem studies of those with schizophrenia. Fewer studies have examined other subunits, and results have been equivocal, except for a reduction in prefrontal NRC2.[22]
The large genome-wide association study mentioned above has supported glutamate abnormalities for schizophrenia, reporting several mutations in genes related to glutamatergic neurotransmission, such as GRIN2A, GRIA1, SRR, and GRM3.[23]
Interneuron dysfunction
Another hypothesis is closely related to the glutamate hypothesis, and involves the dysfunction of inhibitory GABAergic interneurons in the brain.[4][5][6] They are local, and one type, the fast-spiking parvalbumin-positive interneuron, has been suggested to play a key role in schizophrenia pathophysiology.
Early studies have identified decreases in GAD67 mRNA and protein in post-mortem brains from those with schizophrenia compared to controls.[24] These reductions were found in only a subset of cortical interneurons. Furthermore, GAD67 mRNA was completely undetectable in a subset of interneurons also expressing parvalbumin. Levels of parvalbumin protein and mRNA were also found to be lower in various regions in the brain. Actual numbers of parvalbumin interneurons have been found to be unchanged in these studies, however, except for a single study showing a decrease in parvalbumin interneurons in the hippocampus.[25] Finally, excitatory synapse density is lower selectively on parvalbumin interneurons in schizophrenia and predicts the activity-dependent down-regulation of parvalbumin and GAD67.[26] Together, this suggests that parvalbumin interneurons are somehow specifically affected in the disease.
Several studies have tried to assess levels in GABA in vivo in those with schizophrenia, but these findings have remained inconclusive.
EEG studies have indirectly also pointed to interneuron dysfunction in schizophrenia (see below).[27] These studies have pointed to abnormalities in oscillatory activity in schizophrenia, particularly in the gamma band (30–80 Hz). Gamma band activity appears to originate from intact functioning parvalbumin-positive interneuron.[28] Together with the post-mortem findings, these EEG abnormalities point to a role for dysfunctional parvalbumin interneurons in schizophrenia.
The largest meta-analysis on copy-number variations (CNVs), structural abnormalities in the form of genetic deletions or duplications, to date for schizophrenia, published in 2015, was the first genetic evidence for the broad involvement of GABAergic neurotransmission.[29]
Myelination abnormalities
Another hypothesis states that abnormalities in myelination are a core pathophysiology of schizophrenia.[30][31][32] This theory originated from structural imaging studies, which found that white matter regions, in addition to grey matter regions, showed volumetric reductions in people with schizophrenia. In addition, gene expression studies have shown abnormalities in myelination and oligodendrocytes in the post-mortem brains. Furthermore, oligodendrocyte numbers appear to be reduced in several post-mortem studies.[33]
It has been suggested that myelination abnormalities could originate from impaired maturation of oligodendrocyte precursor cells,[34] as these have been found to be intact in schizophrenia brains.
Immune system abnormalities
Inflammation and immune system abnormalities are seen to be key mechanisms for the development of schizophrenia.[9] A number of causes and consequences of inflammation have been implicated many of which are stress-related. Evidence suggests that early stress may contribute to the development of schizophrenia through alterations in the functioning of the immune system.[35] Adverse childhood experiences (ACEs) for example can give toxic stress.[36] ACEs and trauma can disrupt the control of immune responses and give rise to lasting inflammatory dysregulation throughout the nervous system.[35] Chronic trauma can promote chronic immune system activation.[37] Persistent systemic inflammation may lead to damage of the peripheral tissue and a subsequent breach of the blood-brain-barrier. If this happens microglia can be activated and cause neuroinflammation.[35] Inflammation can result in oxidative stress in schizophrenia which has damaging consequences for brain cells.[9]
The immune hypothesis is supported by findings of high levels of immune markers in the blood of people with schizophrenia.[38] High levels of immune markers have also been associated with having more severe psychotic symptoms.[39][40] Furthermore, a meta-analysis of genome-wide association studies discovered that 129 out of 136 single-nucleotide polymorphisms significantly associated with schizophrenia were located in the major histocompatibility complex region of the genome.[41]
A systematic review investigating neuroinflammatory markers in post-mortem schizophrenia brains has shown quite some variability, with some studies showing alterations in various markers but others failing to find any differences.[42]
Oxidative stress
Another theory that has gained support is that a large role is played in the disease by oxidative stress.[8][43][44] Redox dysregulation in early development can potentially influence development of different cell types that have been shown to be impaired in the disease.
Oxidative stress has also been indicated through genetic studies into schizophrenia.[45]
Oxidative stress has been shown to affect maturation of oligodendrocytes,[46] the myelinating cell types in the brain, potentially underlying the white matter abnormalities found in the brain (see below).
Furthermore, oxidative stress could also influence the development of GABAergic interneurons,[47] which have also been found to be dysregulated in schizophrenia (see above).
Evidence that oxidative stress and oxidative DNA damage are increased in various tissues of people with schizophrenia has been reviewed by Markkanen et al.[48] The presence of increased oxidative DNA damage may be due, in part, to insufficient repair of such damages. Several studies have linked polymorphisms in DNA repair genes to the development of schizophrenia.[48] In particular, the base excision repair protein XRCC1 has been implicated.[48]
Neuropathology
The most consistent finding in post-mortem examinations of brain tissue is a lack of neurodegenerative lesions or gliosis. Abnormal neuronal organization and orientation (dysplasia) has been observed in the entorhinal cortex, hippocampus, and subcortical white matter, although results are not entirely consistent. A more consistent cytoarchitectural finding is reduced volume of purkinje cells and pyramidal cells in the hippocampus. This is consistent with the observation of decreased presynaptic terminals in the hippocampus, and a reduction in dendritic spines in the prefrontal cortex.[49] The reductions in prefrontal and increase in striatal spine densities seem to be independent of antipsychotic drug use.[50]
GI tract dysfunction
It has been hypothesized that in some people, development of schizophrenia is related to intestinal tract dysfunction such as seen with non-celiac gluten sensitivity or abnormalities in the gut microbiota.[51] A subgroup of persons with schizophrenia present an immune response to gluten differently from that found in people with celiac, with elevated levels of certain serum biomarkers of gluten sensitivity such as anti-gliadin IgG or anti-gliadin IgA antibodies.[52]
A link has been made between the gut microbiota and the development of TRS. The most prevalent cause put forward for TRS is that of mutation in the genes responsible for drug effectiveness. These include liver enzyme genes that control the availability of a drug to brain targets, and genes responsible for the structure and function of these targets. In the colon the bacteria encode a hundred times more genes than exist in the human genome. Only a fraction of ingested drugs reach the colon, having been already exposed to small intestinal bacteria, and absorbed in the portal circulation. This small fraction is then subject to the metabolic action of many communities of bacteria. Activation of the drug depends on the composition and enzymes of the bacteria and of the specifics of the drug, and therefore a great deal of individual variation can affect both the usefulness of the drug and its tolerability. It is suggested that parenteral administration of antipsychotics would bypass the gut and be more successful in overcoming TRS. The composition of gut microbiota is variable between individuals, but they are seen to remain stable. However, phyla can change in response to many factors including ageing, diet, substance use, and medications – especially antibiotics, laxatives, and antipsychotics. In FEP, schizophrenia has been linked to significant changes in the gut microbiota that can predict response to treatment.[53]
Sleep disorders
It has been suggested that sleep problems may be a core component of the pathophysiology of schizophrenia.[54]
Structural abnormalities
Beside theories concerning the functional mechanism underlying the disease, structural findings have been identified as well using a wide range of imaging techniques. Studies have tended to show various subtle average differences in the volume of certain areas of brain structure between people with and without diagnoses of schizophrenia, although it has become increasingly clear that no single pathological neuropsychological or structural neuroanatomic profile exists.[55]
Morphometry
Structural imaging studies have consistently reported differences in the size and structure of certain brain areas in schizophrenia.
The largest combined neuroimaging study with over 2000 subjects and 2500 controls focused on subcortical changes.[56] Volumetric increases were found in the lateral ventricles (+18%), caudate nucleus and pallidum, and extensive decreases in the hippocampus (-4%), thalamus, amygdala and nucleus accumbens. Together, this indicates that extensive changes do occur in the brains of people with schizophrenia.
A 2006 meta-analysis of MRI studies found that whole brain and hippocampal volume are reduced and that ventricular volume is increased in those with a first psychotic episode relative to healthy controls. The average volumetric changes in these studies are however close to the limit of detection by MRI methods, so it remains to be determined whether schizophrenia is a neurodegenerative process that begins at about the time of symptom onset, or whether it is better characterised as a neurodevelopmental process that produces abnormal brain volumes at an early age.[57] In first episode psychosis typical antipsychotics like haloperidol were associated with significant reductions in gray matter volume, whereas atypical antipsychotics like olanzapine were not.[58] Studies in non-human primates found gray and white matter reductions for both typical and atypical antipsychotics.[59]
Abnormal findings in the prefrontal cortex, temporal cortex and anterior cingulate cortex are found before the first onset of schizophrenia symptoms. These regions are the regions of structural deficits found in schizophrenia and first-episode subjects.[60] Positive symptoms, such as thoughts of being persecuted, were found to be related to the medial prefrontal cortex, amygdala, and hippocampus region. Negative symptoms were found to be related to the ventrolateral prefrontal cortex and ventral striatum.[60]
Ventricular and third ventricle enlargement, abnormal functioning of the amygdala, hippocampus, parahippocampal gyrus, neocortical temporal lobe regions, frontal lobe, prefrontal gray matter, orbitofrontal areas, parietal lobs abnormalities and subcortical abnormalities including the cavum septi pellucidi, basal ganglia, corpus callosum, thalamus and cerebellar abnormalities. Such abnormalities usually present in the form of loss of volume.[61]
Most schizophrenia studies have found average reduced volume of the left medial temporal lobe and left superior temporal gyrus, and half of studies have revealed deficits in certain areas of the frontal gyrus, parahippocampal gyrus and temporal gyrus.[62] However, at variance with some findings in individuals with chronic schizophrenia significant group differences of temporal lobe and amygdala volumes are not shown in first-episode people on average.[63]
Finally, MRI studies utilizing modern cortical surface reconstruction techniques have shown widespread reduction in cerebral cortical thickness (i.e., "cortical thinning") in frontal and temporal regions[64][65] and somewhat less widespread cortical thinning in occipital and parietal regions[65] in people with schizophrenia, relative to healthy control subjects. Moreover, one study decomposed cortical volume into its constituent parts, cortical surface area and cortical thickness, and reported widespread cortical volume reduction in schizophrenia, mainly driven by cortical thinning, but also reduced cortical surface area in smaller frontal, temporal, parietal and occipital cortical regions.[66]
CT scans of the brains of people with schizophrenia show several pathologies. The brain ventricles are enlarged as compared to normal brains. The ventricles hold cerebrospinal fluid (CSF) and enlarged ventricles indicate a loss of brain volume. Additionally, the brains have widened sulci as compared to normal brains, also with increased CSF volumes and reduced brain volume.[61][67]
Using machine learning, two neuroanatomical subtypes of schizophrenia have been described. Subtype 1 shows widespread low grey matter volumes, particularly in the thalamus, nucleus accumbens, medial temporal, medial prefrontal, frontal, and insular cortices. Subtype 2 shows increased volume in the basal ganglia and internal capsule, with otherwise normal brain volume.[68]
White matter
Diffusion tensor imaging (DTI) allows for the investigation of white matter more closely than traditional MRI.[61] Over 300 DTI imaging studies have been published examining white matter abnormalities in schizophrenia.[69][70] Although quite some variation has been found pertaining to the specific regions affected, the general consensus states a reduced fractional anisotropy in brains from people with schizophrenia versus controls. Importantly, these differences between subjects and controls could potentially be attributed to lifestyle effects, medication effects etc. Other studies have looked at people with first-episode schizophrenia that have never received any medication, so-called medication-naive subjects. These studies, although few in number, also found reduced fractional anisotropy in subject brains compared to control brains. As with earlier findings, abnormalities can be found throughout the brain, although the corpus callosum seemed to be most commonly effected.
Functional abnormalities
During executive function tasks in people with schizophrenia, studies using functional magnetic resonance imaging (fMRI) demonstrated decreased activity relative to controls in the bilateral dorsolateral prefrontal cortex (dlPFC), right anterior cingulate cortex (ACC), and left mediodorsal nucleus of the thalamus. Increased activation was observed in the left ACC and left inferior parietal lobe.[71] During emotional processing tasks, reduced activations have been observed in the medial prefrontal cortex, ACC, dlPFC and amygdala.[72] A meta-analysis of facial emotional processing observed decreased activation in the amygdala, parahippocampus, lentiform nuclei, fusiform gyrus and right superior frontal gyrus, as well as increased activation in the left insula.[73]
One meta-analysis of fMRI during acute auditory verbal hallucinations has reported increased activations in areas implicated in language, including the bilateral inferior frontal and post central gyri, as well as the left parietal operculum.[74] Another meta analysis during both visual and auditory verbal hallucinations, replicated the findings in the inferior frontal and postcentral gyri during auditory verbal hallucinations, and also observed hippocampal, superior temporal, insular and medial prefrontal activations. Visual hallucinations were reported to be associated with increased activations in the secondary and associate visual cortices.[75]
PET
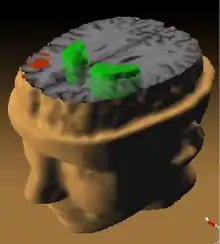
PET scan findings in people with schizophrenia indicate cerebral blood flow decreases in the left parahippocampal region. A reduced ability to metabolize glucose in the thalamus and frontal cortex is also shown. PET scans show developmental abnormality in the medial part of the left temporal lobe, and the limbic, and frontal systems. PET scans show that thought disorders stem from increased blood flow in the frontal and temporal regions while delusions and hallucinations were associated with reduced flow in the cingulate, left frontal, and temporal areas. PET scans carried out during active auditory hallucinations revealed increased blood flow in the thalamus, left hippocampus, right striatum, parahippocampus, orbitofrontal, and cingulate areas.[61]
In addition, a decrease in NAA uptake has been reported in the hippocampus and both the grey and white matter of the prefrontal cortex. NAA may be an indicator of neural activity of number of viable neurons. However, given methodological limitations and variance it is not possible to use this as a diagnostic method.[77] Decreased prefrontal cortex connectivity has also been observed.[78] DOPA PET studies have confirmed an altered synthesis capacity of dopamine in the nigrostriatal system demonstrating a dopaminergic dysregulation.[79][12]
References
- 1 2 van Os J, Kapur S (August 2009). "Schizophrenia". Lancet. 374 (9690): 635–45. doi:10.1016/S0140-6736(09)60995-8. PMID 19700006. S2CID 208792724.
- ↑ Insel TR (November 2010). "Rethinking schizophrenia". Nature. 468 (7321): 187–93. Bibcode:2010Natur.468..187I. doi:10.1038/nature09552. PMID 21068826. S2CID 4416517.
- ↑ Elert E (April 2014). "Aetiology: Searching for schizophrenia's roots". Nature. 508 (7494): S2–3. Bibcode:2014Natur.508S...2E. doi:10.1038/508S2a. PMID 24695332.
- 1 2 Marín O (January 2012). "Interneuron dysfunction in psychiatric disorders". Nature Reviews. Neuroscience. 13 (2): 107–20. doi:10.1038/nrn3155. PMID 22251963. S2CID 205507186.
- 1 2 Gonzalez-Burgos G, Cho RY, Lewis DA (June 2015). "Alterations in cortical network oscillations and parvalbumin neurons in schizophrenia". Biological Psychiatry. 77 (12): 1031–40. doi:10.1016/j.biopsych.2015.03.010. PMC 4444373. PMID 25863358.
- 1 2 Pittman-Polletta BR, Kocsis B, Vijayan S, Whittington MA, Kopell NJ (June 2015). "Brain rhythms connect impaired inhibition to altered cognition in schizophrenia". Biological Psychiatry. 77 (12): 1020–30. doi:10.1016/j.biopsych.2015.02.005. PMC 4444389. PMID 25850619.
- ↑ Feigenson KA, Kusnecov AW, Silverstein SM (January 2014). "Inflammation and the two-hit hypothesis of schizophrenia". Neuroscience and Biobehavioral Reviews. 38: 72–93. doi:10.1016/j.neubiorev.2013.11.006. PMC 3896922. PMID 24247023.
- 1 2 Steullet P, Cabungcal JH, Monin A, Dwir D, O'Donnell P, Cuenod M, Do KQ (September 2016). "Redox dysregulation, neuroinflammation, and NMDA receptor hypofunction: A "central hub" in schizophrenia pathophysiology?". Schizophrenia Research. 176 (1): 41–51. doi:10.1016/j.schres.2014.06.021. PMC 4282982. PMID 25000913.
- 1 2 3 Leza JC, García-Bueno B, Bioque M, Arango C, Parellada M, Do K, O'Donnell P, Bernardo M (August 2015). "Inflammation in schizophrenia: A question of balance". Neuroscience and Biobehavioral Reviews. 55: 612–26. doi:10.1016/j.neubiorev.2015.05.014. PMID 26092265. S2CID 35480356.
- ↑ Howes OD, Kambeitz J, Kim E, Stahl D, Slifstein M, Abi-Dargham A, Kapur S (August 2012). "The nature of dopamine dysfunction in schizophrenia and what this means for treatment". Archives of General Psychiatry. 69 (8): 776–86. doi:10.1001/archgenpsychiatry.2012.169. PMC 3730746. PMID 22474070.
- ↑ Kambeitz J, Abi-Dargham A, Kapur S, Howes OD (June 2014). "Alterations in cortical and extrastriatal subcortical dopamine function in schizophrenia: systematic review and meta-analysis of imaging studies". The British Journal of Psychiatry. 204 (6): 420–9. doi:10.1192/bjp.bp.113.132308. PMID 25029687.
- 1 2 Weinstein JJ, Chohan MO, Slifstein M, Kegeles LS, Moore H, Abi-Dargham A (January 2017). "Pathway-Specific Dopamine Abnormalities in Schizophrenia". Biological Psychiatry. 81 (1): 31–42. doi:10.1016/j.biopsych.2016.03.2104. PMC 5177794. PMID 27206569.
- ↑ Chun S, Westmoreland JJ, Bayazitov IT, Eddins D, Pani AK, Smeyne RJ, Yu J, Blundon JA, Zakharenko SS (June 2014). "Specific disruption of thalamic inputs to the auditory cortex in schizophrenia models". Science. 344 (6188): 1178–82. Bibcode:2014Sci...344.1178C. doi:10.1126/science.1253895. PMC 4349506. PMID 24904170.
- ↑ Kapur S (January 2003). "Psychosis as a state of aberrant salience: a framework linking biology, phenomenology, and pharmacology in schizophrenia". The American Journal of Psychiatry. 160 (1): 13–23. doi:10.1176/appi.ajp.160.1.13. PMID 12505794.
- ↑ Takahashi H, Higuchi M, Suhara T (May 2006). "The role of extrastriatal dopamine D2 receptors in schizophrenia". Biological Psychiatry. 59 (10): 919–28. doi:10.1016/j.biopsych.2006.01.022. PMID 16682269. S2CID 20866362.
- ↑ Corlett PR, Taylor JR, Wang XJ, Fletcher PC, Krystal JH (November 2010). "Toward a neurobiology of delusions". Progress in Neurobiology. 92 (3): 345–69. doi:10.1016/j.pneurobio.2010.06.007. PMC 3676875. PMID 20558235.
- ↑ Konradi C, Heckers S (February 2003). "Molecular aspects of glutamate dysregulation: implications for schizophrenia and its treatment". Pharmacology & Therapeutics. 97 (2): 153–79. doi:10.1016/S0163-7258(02)00328-5. PMC 4203361. PMID 12559388.
- ↑ Lahti AC, Weiler MA, Tamara Michaelidis BA, Parwani A, Tamminga CA (October 2001). "Effects of ketamine in normal and schizophrenic volunteers". Neuropsychopharmacology. 25 (4): 455–67. doi:10.1016/S0893-133X(01)00243-3. PMID 11557159.
- ↑ Coyle JT, Tsai G, Goff D (November 2003). "Converging evidence of NMDA receptor hypofunction in the pathophysiology of schizophrenia". Annals of the New York Academy of Sciences. 1003 (1): 318–27. Bibcode:2003NYASA1003..318C. doi:10.1196/annals.1300.020. PMID 14684455. S2CID 1358620.
- ↑ Tuominen HJ, Tiihonen J, Wahlbeck K (January 2005). "Glutamatergic drugs for schizophrenia: a systematic review and meta-analysis". Schizophrenia Research. 72 (2–3): 225–34. doi:10.1016/j.schres.2004.05.005. PMID 15560967. S2CID 22407838.
- ↑ Weickert CS, Fung SJ, Catts VS, Schofield PR, Allen KM, Moore LT, Newell KA, Pellen D, Huang XF, Catts SV, Weickert TW (November 2013). "Molecular evidence of N-methyl-D-aspartate receptor hypofunction in schizophrenia". Molecular Psychiatry. 18 (11): 1185–92. doi:10.1038/mp.2012.137. PMC 3807670. PMID 23070074.
- ↑ Catts VS, Lai YL, Weickert CS, Weickert TW, Catts SV (April 2016). "A quantitative review of the postmortem evidence for decreased cortical N-methyl-D-aspartate receptor expression levels in schizophrenia: How can we link molecular abnormalities to mismatch negativity deficits?". Biological Psychology. 116: 57–67. doi:10.1016/j.biopsycho.2015.10.013. PMID 26549579.
- ↑ Schizophrenia Working Group of the Psychiatric Genomics Consortium (July 2014). "Biological insights from 108 schizophrenia-associated genetic loci". Nature. 511 (7510): 421–7. Bibcode:2014Natur.511..421S. doi:10.1038/nature13595. PMC 4112379. PMID 25056061.
- ↑ Gonzalez-Burgos G, Hashimoto T, Lewis DA (August 2010). "Alterations of cortical GABA neurons and network oscillations in schizophrenia". Current Psychiatry Reports. 12 (4): 335–44. doi:10.1007/s11920-010-0124-8. PMC 2919752. PMID 20556669.
- ↑ Konradi C, Yang CK, Zimmerman EI, Lohmann KM, Gresch P, Pantazopoulos H, Berretta S, Heckers S (September 2011). "Hippocampal interneurons are abnormal in schizophrenia". Schizophrenia Research. 131 (1–3): 165–73. doi:10.1016/j.schres.2011.06.007. PMC 3159834. PMID 21745723.
- ↑ Chung DW, Fish KN, Lewis DA (November 2016). "Pathological Basis for Deficient Excitatory Drive to Cortical Parvalbumin Interneurons in Schizophrenia". The American Journal of Psychiatry. 173 (11): 1131–1139. doi:10.1176/appi.ajp.2016.16010025. PMC 5089927. PMID 27444795.
- ↑ Senkowski D, Gallinat J (June 2015). "Dysfunctional prefrontal gamma-band oscillations reflect working memory and other cognitive deficits in schizophrenia". Biological Psychiatry. 77 (12): 1010–9. doi:10.1016/j.biopsych.2015.02.034. PMID 25847179. S2CID 206104940.
- ↑ Sohal VS, Zhang F, Yizhar O, Deisseroth K (June 2009). "Parvalbumin neurons and gamma rhythms enhance cortical circuit performance". Nature. 459 (7247): 698–702. Bibcode:2009Natur.459..698S. doi:10.1038/nature07991. PMC 3969859. PMID 19396159.
- ↑ Pocklington AJ, Rees E, Walters JT, Han J, Kavanagh DH, Chambert KD, Holmans P, Moran JL, McCarroll SA, Kirov G, O'Donovan MC, Owen MJ (June 2015). "Novel Findings from CNVs Implicate Inhibitory and Excitatory Signaling Complexes in Schizophrenia". Neuron. 86 (5): 1203–14. doi:10.1016/j.neuron.2015.04.022. PMC 4460187. PMID 26050040.
- ↑ Cassoli JS, Guest PC, Malchow B, Schmitt A, Falkai P, Martins-de-Souza D (2015-01-01). "Disturbed macro-connectivity in schizophrenia linked to oligodendrocyte dysfunction: from structural findings to molecules". npj Schizophrenia. 1: 15034. doi:10.1038/npjschz.2015.34. PMC 4849457. PMID 27336040.
- ↑ Mighdoll MI, Tao R, Kleinman JE, Hyde TM (January 2015). "Myelin, myelin-related disorders, and psychosis". Schizophrenia Research. 161 (1): 85–93. doi:10.1016/j.schres.2014.09.040. PMID 25449713. S2CID 23435472.
- ↑ Haroutunian V, Katsel P, Roussos P, Davis KL, Altshuler LL, Bartzokis G (November 2014). "Myelination, oligodendrocytes, and serious mental illness". Glia. 62 (11): 1856–77. doi:10.1002/glia.22716. PMID 25056210. S2CID 571262.
- ↑ Stedehouder J, Kushner SA (January 2017). "Myelination of parvalbumin interneurons: a parsimonious locus of pathophysiological convergence in schizophrenia". Molecular Psychiatry. 22 (1): 4–12. doi:10.1038/mp.2016.147. PMC 5414080. PMID 27646261.
- ↑ Mauney SA, Pietersen CY, Sonntag KC, Woo TW (December 2015). "Differentiation of oligodendrocyte precursors is impaired in the prefrontal cortex in schizophrenia". Schizophrenia Research. 169 (1–3): 374–380. doi:10.1016/j.schres.2015.10.042. PMC 4681621. PMID 26585218.
- 1 2 3 Nettis MA, Pariante CM, Mondelli V (2020). "Early-Life Adversity, Systemic Inflammation and Comorbid Physical and Psychiatric Illnesses of Adult Life". Current Topics in Behavioral Neurosciences. 44: 207–225. doi:10.1007/7854_2019_89. ISBN 978-3-030-39140-9. PMID 30895531.
- ↑ Pearce J, Murray C, Larkin W (July 2019). "Childhood adversity and trauma: experiences of professionals trained to routinely enquire about childhood adversity". Heliyon. 5 (7): e01900. doi:10.1016/j.heliyon.2019.e01900. PMC 6658729. PMID 31372522.
- ↑ Chase KA, Melbourne JK, Rosen C (March 2019). "Traumagenics: At the intersect of childhood trauma, immunity and psychosis". Psychiatry Research. 273: 369–377. doi:10.1016/j.psychres.2018.12.097. PMID 30682559. S2CID 56177674.
- ↑ Hope S, Melle I, Aukrust P, Steen NE, Birkenaes AB, Lorentzen S, Agartz I, Ueland T, Andreassen OA (November 2009). "Similar immune profile in bipolar disorder and schizophrenia: selective increase in soluble tumor necrosis factor receptor I and von Willebrand factor" (PDF). Bipolar Disorders. 11 (7): 726–34. doi:10.1111/j.1399-5618.2009.00757.x. hdl:10852/34620. PMID 19839997.
- ↑ Drexhage RC, Knijff EM, Padmos RC, Heul-Nieuwenhuijzen L, Beumer W, Versnel MA, Drexhage HA (January 2010). "The mononuclear phagocyte system and its cytokine inflammatory networks in schizophrenia and bipolar disorder". Expert Review of Neurotherapeutics. 10 (1): 59–76. doi:10.1586/ern.09.144. PMID 20021321. S2CID 207220109.
- ↑ Hope S, Ueland T, Steen NE, Dieset I, Lorentzen S, Berg AO, Agartz I, Aukrust P, Andreassen OA (April 2013). "Interleukin 1 receptor antagonist and soluble tumor necrosis factor receptor 1 are associated with general severity and psychotic symptoms in schizophrenia and bipolar disorder". Schizophrenia Research. 145 (1–3): 36–42. doi:10.1016/j.schres.2012.12.023. PMID 23403415.
- ↑ Schizophrenia Psychiatric Genome-Wide Association Study (GWAS) Consortium (September 2011). "Genome-wide association study identifies five new schizophrenia loci". Nature Genetics. 43 (10): 969–76. doi:10.1038/ng.940. PMC 3303194. PMID 21926974.
- ↑ Trépanier MO, Hopperton KE, Mizrahi R, Mechawar N, Bazinet RP (August 2016). "Postmortem evidence of cerebral inflammation in schizophrenia: a systematic review". Molecular Psychiatry. 21 (8): 1009–26. doi:10.1038/mp.2016.90. PMC 4960446. PMID 27271499.
- ↑ Emiliani FE, Sedlak TW, Sawa A (May 2014). "Oxidative stress and schizophrenia: recent breakthroughs from an old story". Current Opinion in Psychiatry. 27 (3): 185–90. doi:10.1097/YCO.0000000000000054. PMC 4054867. PMID 24613987.
- ↑ Hardingham GE, Do KQ (February 2016). "Linking early-life NMDAR hypofunction and oxidative stress in schizophrenia pathogenesis" (PDF). Nature Reviews. Neuroscience. 17 (2): 125–34. doi:10.1038/nrn.2015.19. PMID 26763624. S2CID 27164937.
- ↑ Kushima I, Aleksic B, Nakatochi M, Shimamura T, Shiino T, Yoshimi A, et al. (March 2017). "High-resolution copy number variation analysis of schizophrenia in Japan". Molecular Psychiatry. 22 (3): 430–440. doi:10.1038/mp.2016.88. PMID 27240532. S2CID 3605506.
- ↑ Monin A, Baumann PS, Griffa A, Xin L, Mekle R, Fournier M, et al. (July 2015). "Glutathione deficit impairs myelin maturation: relevance for white matter integrity in schizophrenia patients". Molecular Psychiatry. 20 (7): 827–38. doi:10.1038/mp.2014.88. PMID 25155877. S2CID 15364819.
- ↑ Cabungcal JH, Steullet P, Kraftsik R, Cuenod M, Do KQ (March 2013). "Early-life insults impair parvalbumin interneurons via oxidative stress: reversal by N-acetylcysteine". Biological Psychiatry. 73 (6): 574–82. doi:10.1016/j.biopsych.2012.09.020. PMID 23140664. S2CID 24651769.
- 1 2 3 Markkanen E, Meyer U, Dianov GL (June 2016). "DNA Damage and Repair in Schizophrenia and Autism: Implications for Cancer Comorbidity and Beyond". Int J Mol Sci. 17 (6): 856. doi:10.3390/ijms17060856. PMC 4926390. PMID 27258260.
- ↑ Harrison PJ (December 2000). "Postmortem studies in schizophrenia". Dialogues in Clinical Neuroscience. 2 (4): 349–57. doi:10.31887/DCNS.2000.2.4/pharrison. PMC 3181616. PMID 22033474.
- ↑ Glausier JR, Lewis DA (October 2013). "Dendritic spine pathology in schizophrenia". Neuroscience. 251: 90–107. doi:10.1016/j.neuroscience.2012.04.044. PMC 3413758. PMID 22546337.
- ↑ Nemani K, Hosseini Ghomi R, McCormick B, Fan X (January 2015). "Schizophrenia and the gut-brain axis". Progress in Neuro-Psychopharmacology & Biological Psychiatry. 56: 155–60. doi:10.1016/j.pnpbp.2014.08.018. PMID 25240858. S2CID 32315340.
- ↑ Lachance LR, McKenzie K (February 2014). "Biomarkers of gluten sensitivity in patients with non-affective psychosis: a meta-analysis". Schizophrenia Research (Review). 152 (2–3): 521–7. doi:10.1016/j.schres.2013.12.001. PMID 24368154. S2CID 26792210.
- ↑ Seeman MV (March 2020). "The Gut Microbiome and Treatment-Resistance in Schizophrenia". The Psychiatric Quarterly. 91 (1): 127–136. doi:10.1007/s11126-019-09695-4. PMID 31781943. S2CID 208329435.
- ↑ Pocivavsek, A; Rowland, LM (13 January 2018). "Basic Neuroscience Illuminates Causal Relationship Between Sleep and Memory: Translating to Schizophrenia". Schizophrenia Bulletin. 44 (1): 7–14. doi:10.1093/schbul/sbx151. PMC 5768044. PMID 29136236.
- ↑ Flashman LA, Green MF (March 2004). "Review of cognition and brain structure in schizophrenia: profiles, longitudinal course, and effects of treatment". The Psychiatric Clinics of North America. 27 (1): 1–18, vii. doi:10.1016/S0193-953X(03)00105-9. PMID 15062627.
- ↑ van Erp TG, Hibar DP, Rasmussen JM, Glahn DC, Pearlson GD, Andreassen OA, et al. (April 2016). "Subcortical brain volume abnormalities in 2028 individuals with schizophrenia and 2540 healthy controls via the ENIGMA consortium". Molecular Psychiatry. 21 (4): 547–53. doi:10.1038/mp.2015.63. PMC 4668237. PMID 26033243.
- ↑ Steen RG, Mull C, McClure R, Hamer RM, Lieberman JA (June 2006). "Brain volume in first-episode schizophrenia: systematic review and meta-analysis of magnetic resonance imaging studies". The British Journal of Psychiatry. 188 (6): 510–8. doi:10.1192/bjp.188.6.510. PMID 16738340.
- ↑ Lieberman JA, Bymaster FP, Meltzer HY, Deutch AY, Duncan GE, Marx CE, Aprille JR, Dwyer DS, Li XM, Mahadik SP, Duman RS, Porter JH, Modica-Napolitano JS, Newton SS, Csernansky JG (September 2008). "Antipsychotic drugs: comparison in animal models of efficacy, neurotransmitter regulation, and neuroprotection". Pharmacological Reviews. 60 (3): 358–403. doi:10.1124/pr.107.00107. PMC 4821196. PMID 18922967.
- ↑ DeLisi LE (March 2008). "The concept of progressive brain change in schizophrenia: implications for understanding schizophrenia". Schizophrenia Bulletin. 34 (2): 312–21. doi:10.1093/schbul/sbm164. PMC 2632405. PMID 18263882.
- 1 2 Jung WH, Jang JH, Byun MS, An SK, Kwon JS (December 2010). "Structural brain alterations in individuals at ultra-high risk for psychosis: a review of magnetic resonance imaging studies and future directions". Journal of Korean Medical Science. 25 (12): 1700–9. doi:10.3346/jkms.2010.25.12.1700. PMC 2995221. PMID 21165282.
- 1 2 3 4 Shenton ME, Dickey CC, Frumin M, McCarley RW (April 2001). "A review of MRI findings in schizophrenia". Schizophrenia Research. 49 (1–2): 1–52. doi:10.1016/s0920-9964(01)00163-3. PMC 2812015. PMID 11343862.
- ↑ Honea R, Crow TJ, Passingham D, Mackay CE (December 2005). "Regional deficits in brain volume in schizophrenia: a meta-analysis of voxel-based morphometry studies". The American Journal of Psychiatry. 162 (12): 2233–45. doi:10.1176/appi.ajp.162.12.2233. PMID 16330585.
- ↑ Vita A, De Peri L, Silenzi C, Dieci M (February 2006). "Brain morphology in first-episode schizophrenia: a meta-analysis of quantitative magnetic resonance imaging studies". Schizophrenia Research. 82 (1): 75–88. doi:10.1016/j.schres.2005.11.004. PMID 16377156. S2CID 28037606.
- ↑ Kuperberg GR, Broome MR, McGuire PK, David AS, Eddy M, Ozawa F, Goff D, West WC, Williams SC, van der Kouwe AJ, Salat DH, Dale AM, Fischl B (September 2003). "Regionally localized thinning of the cerebral cortex in schizophrenia". Archives of General Psychiatry. 60 (9): 878–88. doi:10.1001/archpsyc.60.9.878. PMID 12963669.
- 1 2 Rimol LM, Hartberg CB, Nesvåg R, Fennema-Notestine C, Hagler DJ, Pung CJ, Jennings RG, Haukvik UK, Lange E, Nakstad PH, Melle I, Andreassen OA, Dale AM, Agartz I (July 2010). "Cortical thickness and subcortical volumes in schizophrenia and bipolar disorder". Biological Psychiatry. 68 (1): 41–50. doi:10.1016/j.biopsych.2010.03.036. PMID 20609836. S2CID 7718134.
- ↑ Rimol LM, Nesvåg R, Hagler DJ, Bergmann O, Fennema-Notestine C, Hartberg CB, Haukvik UK, Lange E, Pung CJ, Server A, Melle I, Andreassen OA, Agartz I, Dale AM (March 2012). "Cortical volume, surface area, and thickness in schizophrenia and bipolar disorder". Biological Psychiatry. 71 (6): 552–60. doi:10.1016/j.biopsych.2011.11.026. PMID 22281121. S2CID 27413169.
- ↑ Ropper AH, Brown RH (2005). Adams and Victor's Principles of Neurology (8th ed.). New York: McGraw-Hill. p. 1324. ISBN 0-07-141620-X.
- ↑ Chand GB, Dwyer DB, Erus G, et al. (27 February 2020). "Two distinct neuroanatomical subtypes of schizophrenia revealed using machine learning". Brain: A Journal of Neurology. 143 (3): 1027–1038. doi:10.1093/brain/awaa025. PMC 7089665. PMID 32103250.
- ↑ Fitzsimmons J, Kubicki M, Shenton ME (March 2013). "Review of functional and anatomical brain connectivity findings in schizophrenia". Current Opinion in Psychiatry. 26 (2): 172–87. doi:10.1097/YCO.0b013e32835d9e6a. PMID 23324948. S2CID 24317327.
- ↑ Ellison-Wright I, Bullmore E (March 2009). "Meta-analysis of diffusion tensor imaging studies in schizophrenia". Schizophrenia Research. 108 (1–3): 3–10. doi:10.1016/j.schres.2008.11.021. PMID 19128945. S2CID 39073686.
- ↑ Minzenberg MJ, Laird AR, Thelen S, Carter CS, Glahn DC (August 2009). "Meta-analysis of 41 functional neuroimaging studies of executive function in schizophrenia". Archives of General Psychiatry. 66 (8): 811–22. doi:10.1001/archgenpsychiatry.2009.91. PMC 2888482. PMID 19652121.
- ↑ Taylor SF, Kang J, Brege IS, Tso IF, Hosanagar A, Johnson TD (January 2012). "Meta-analysis of functional neuroimaging studies of emotion perception and experience in schizophrenia". Biological Psychiatry. 71 (2): 136–45. doi:10.1016/j.biopsych.2011.09.007. PMC 3237865. PMID 21993193.
- ↑ Li H, Chan RC, McAlonan GM, Gong QY (September 2010). "Facial emotion processing in schizophrenia: a meta-analysis of functional neuroimaging data". Schizophrenia Bulletin. 36 (5): 1029–39. doi:10.1093/schbul/sbn190. PMC 2930350. PMID 19336391.
- ↑ Kühn S, Gallinat J (June 2012). "Quantitative meta-analysis on state and trait aspects of auditory verbal hallucinations in schizophrenia". Schizophrenia Bulletin. 38 (4): 779–86. doi:10.1093/schbul/sbq152. PMC 3406531. PMID 21177743.
- ↑ Zmigrod L, Garrison JR, Carr J, Simons JS (October 2016). "The neural mechanisms of hallucinations: A quantitative meta-analysis of neuroimaging studies". Neuroscience and Biobehavioral Reviews. 69: 113–23. doi:10.1016/j.neubiorev.2016.05.037. PMID 27473935.
- ↑ Meyer-Lindenberg A, Miletich RS, Kohn PD, Esposito G, Carson RE, Quarantelli M, Weinberger DR, Berman KF (March 2002). "Reduced prefrontal activity predicts exaggerated striatal dopaminergic function in schizophrenia". Nature Neuroscience. 5 (3): 267–71. doi:10.1038/nn804. PMID 11865311. S2CID 26098214.
- ↑ Steen RG, Hamer RM, Lieberman JA (November 2005). "Measurement of brain metabolites by 1H magnetic resonance spectroscopy in patients with schizophrenia: a systematic review and meta-analysis". Neuropsychopharmacology. 30 (11): 1949–62. doi:10.1038/sj.npp.1300850. PMID 16123764.
- ↑ Davis KL, American College of Neuropsychopharmacology (2002). "Chapter 53: Neural Circuitry and the Pathophysiology of Schizophrenia". Neuropsychopharmacology: The Fifth Generation of Progress : an Official Publication of the American College of Neuropsychopharmacology. Lippincott Williams & Wilkins. pp. 731–743. ISBN 9780781728379.
- ↑ Fusar-Poli P, Meyer-Lindenberg A (January 2013). "Striatal presynaptic dopamine in schizophrenia, part II: meta-analysis of [(18)F/(11)C]-DOPA PET studies". Schizophrenia Bulletin. 39 (1): 33–42. doi:10.1093/schbul/sbr180. PMC 3523905. PMID 22282454.
External links
Media related to Mechanisms of schizophrenia at Wikimedia Commons