Tylosaurus | |
---|---|
![]() | |
Mounted reconstruction of the T. proriger "Bunker" specimen (KUVP 5033) | |
Scientific classification ![]() | |
Domain: | Eukaryota |
Kingdom: | Animalia |
Phylum: | Chordata |
Class: | Reptilia |
Order: | Squamata |
Superfamily: | †Mosasauroidea |
Family: | †Mosasauridae |
Clade: | †Russellosaurina |
Subfamily: | †Tylosaurinae |
Genus: | †Tylosaurus Marsh, 1872 |
Type species | |
†Tylosaurus proriger Cope, 1869 | |
Other species | |
Disputed or unpublished
| |
Synonyms | |
List of synonyms
|
Tylosaurus (from the ancient Greek τύλος (tylos) 'protuberance, knob' + Greek σαῦρος (sauros) 'lizard') is a genus of mosasaur, a large, predatory marine reptile closely related to modern monitor lizards and to snakes, from the Late Cretaceous.
Discovery and naming
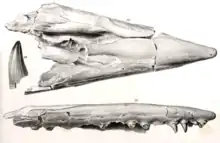
Tylosaurus was the third new genus of mosasaur to be described from North America behind Clidastes and Platecarpus and the first in Kansas.[9] The early history of the genus as a taxon was subject to complications spurred by the infamous rivalry between American paleontologists Edward Drinker Cope and Othniel Charles Marsh during the Bone Wars.[9][10] The type specimen was described by Cope in 1869 based on a fragmentary skull measuring nearly 5 feet (1.5 m) in length and thirteen vertebrae lent to him by Louis Agassiz of the Harvard Museum of Comparative Zoology.[11] The fossil, which remains in the same museum under the catalog number MCZ 4374, was recovered from a deposit of the Niobrara Formation located in the vicinity of Monument Rocks[12] near the Union Pacific Railroad at Fort Hays, Kansas.[13] Cope's first publication of the fossil was very brief and was named Macrosaurus proriger, the genus being a preexisting European mosasaur taxon.[9][11] The specific epithet proriger means "prow-bearing", which is in reference to the specimen's unique prow-like elongated rostrum[14][10] and is derived from the Latin word prōra (prow) and suffix -gero (I bear).[15] In 1870, Cope published a more thorough description of MCZ 4374. Without explanation, he moved the species into another European genus Liodon and declared his original Macrosaurus proriger a synonym.[9][13]
In 1872, Marsh argued that Liodon proriger is taxonomically distinct from the European genus and must be assigned a new one. For this, he erected the genus Rhinosaurus, which means "nose lizard" and is a portmanteau derived from the Ancient Greek words ῥίς (rhī́s, meaning "nose") and σαῦρος (saûros, meaning "lizard"). Marsh also described a third species based on a partial skeleton he collected near the southern portion of the Smoky Hill River[16] that is now in the Yale Peabody Museum as YPM 1268,[17][18] which Marsh named Rhinosaurus micromus.[16][18] Cope responded by arguing that Rhinosaurus was already a preoccupied synonym of Liodon. He disagreed with Marsh's arguments but proposed that in case Marsh was indeed correct, the genus name Rhamphosaurus should be used.[19] Marsh later discovered that the taxon Rhamphosaurus was preoccupied as a genus of lizard named in 1843. As a result, he suggested a move to a newly erected genus named Tylosaurus.[20] This name means "knob lizard" in another reference to the elongated rostrum characteristic of the genus. It is derived from the Latin tylos (knob) and Ancient Greek σαῦρος.[10] Despite coining the new genus, Marsh never formally transferred the Rhinosaurus species to Tylosaurus; this was first done in 1873 by Joseph Leidy by transferring Rhinosaurus proriger to Tylosaurus.[21][22] Rhinosaurus micromus was formally transferred to the same genus in 1894 by John Campbell Merriam.[23]
Description
Size
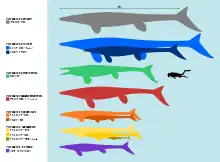
Tylosaurus was one of the largest known mosasaurs. The largest well-known specimen, a skeleton of T. proriger from the University of Kansas Natural History Museum nicknamed "Bunker" (KUVP 5033), has been estimated to measure between 12–15.8 meters (39–52 ft) long.[21][24] A fragmentary skeleton of another T. proriger from the Sternberg Museum of Natural History (FHSM VP-2496) may have been even larger; Everhart estimated the specimen to come from a 14 meters (46 ft) individual[25] compared to his 12 meters (39 ft) estimate for Bunker.[26] The genus exhibits Cope's rule, in which its body size has been observed to generally increase over geologic time.[21] In North America, the earliest representatives of Tylosaurus during the Turonian[27] and Coniacian (90-86 mya), which included early T. nepaeolicus and its precursors, typically measured 5–7 meters (16–23 ft) long[21] and weighed between 200–500 kilograms (440–1,100 lb).[28] During the Santonian (86-83 mya), T. nepaeolicus and newly-appearing T. proriger were 8–9 meters (26–30 ft) long[21] and weighed around 1,100 kilograms (2,400 lb).[29] By the Early Campanian, T. proriger attained lengths of 13–14 meters (43–46 ft).[30] Everhart speculated that because mosasaurs continuously grew throughout their lifetime, it would have been possible for some extremely old Tylosaurus individuals to reach 20 meters (66 ft) in absolute maximum length. However, he stressed the lack of fossil evidence suggesting such sizes and the odds against any being preserved.[31]
Other Campanian-Maastrichtian species were similarly large. The most recent maximum estimate for T. bernardi is 12.2 meters (40 ft) by Lindgren (2005); historically the species was erroneously estimated at even larger sizes of 15–17 meters (49–56 ft).[32] A reconstruction of T. saskatchwanensis by the Royal Saskatchewan Museum estimated a total length of over 9.75 meters (32.0 ft).[33] A mounted skeleton of T. pembinensis nicknamed "Bruce" at the Canadian Fossil Discovery Centre measures at 13.05 meters (42.8 ft) long and was awarded a Guinness World Records for "Largest mosasaur on display" in 2014.[34] However, the skeleton was assembled for display prior[35] to Bullard and Caldwell (2010)'s reassessment that found the species' number of vertebrae to be exaggerated.[36] T. 'borealis' is estimated at 6.5–8 meters (21–26 ft) in total length.[37]
Skull
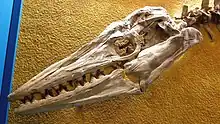
The largest measured skull of Tylosaurus is Bunker's, estimated at 1.7 meters (5.6 ft) long.[38] Depending on age and individual variation,[38] it made up between 13-14% of the total skeleton length.[39] The head was strongly conical and the snout proportionally longer than many mosasaurs with exceptions like Ectenosaurus.[40]
Jaws and teeth
The upper jawbones include the premaxilla and maxilla, while the sole tooth-bearing lower jawbone is the dentary. Together, their teeth forms the marginal dentition. The jaws were elongate; the dentary is between 56-60% of total length of the entire lower jaw in adult T. nepaeolicus and T. proriger,[38] about 55% in T. pembinensis,[41] and 62% in T. saskatchwanensis.[42] It is about 55% of the total skull length, which is slightly shorter than the lower jaw, in T. bernardi.[32] The dentary is robust and strong, though not as strongly built as Mosasaurus, Prognathodon, or Plesiotylosaurus.[43] The jawline ranges from flat[6] to slightly concave.[36][44] A small dorsal ridge appears right ahead of the frontmost dentary tooth in at least mature individuals of T. nepaeolicus and T. proriger.[38] The maxilla in at least T. bernardi is long and slender.[45] Like all mosasaurs, Tylosaurus also had teeth in the pterygoid bone, the rear bone of the palate.[46] The pterygoid teeth probably functioned to perform ratchet feeding, in which the teeth holds prey in place as the mosasaur's lower jaw slides back and forth in a streoptostylic manner enabled through a modified jaw joint.[47] In each half of the skull, Tylosaurus had 2 premaxillary teeth and 12 to 13 maxillary teeth in the upper jaw, 13 dentary teeth on the lower jaw, and 10 to 11 pterygoid teeth.[6] The genus displayed a mostly homodont dentition, meaning that its teeth were minimally different in size and shape.[48][36][3] Only the pterygoid teeth significantly differed from the rest.[49]
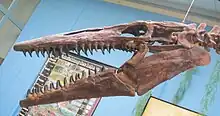
The marginal dentition of most species is adapted for cutting large marine vertebrates,[37][50] while those in T. ivoensis and T. gaudryi appear more optimized for piercing or smashing prey,[5] and T. 'borealis' in both piercing and cutting.[37] Marginal teeth are triangular with a slight recurve towards the back of the jaws so that the lingual (tongue-facing) side forms a U-shaped curve.[48] From top view, they are compressed at the lingual and labial (front lip facing) sides to form an oval-like shape.[51][42] Teeth of immature T. proriger are initially compressed, but become conical in adulthood.[49][52][53] Compression were initially believed to occur at the lingual and buccal (side tongue facing) sides in T. bernardi,[49] but the latest examination by Jiménez-Huidobro and Caldwell (2016) found its teeth to be compressed lingually and labially.[45] Cutting edges are finely serrated with small denticles[6][45][51] except in juvenile T. nepaeolicus.[51] In T. pembinensis, they are faint.[36] The teeth generally hold a pair of cutting edges facing the front and back of the jaws, but some front teeth may have only one on the front side.[51][42] The placement of cutting edges if paired are not always equal; in at least T. proriger, T. ivoensis, T. gaudryi,[49] and T. pembinensis, they are positioned such that the surface area of the tooth's lingual side is greater than the buccal.[36] Both sides are always balanced in area in T. bernardi.[49] The enamel surface is lined with thin fine ridges called striations that run vertically from the tooth's base. Surface is also either smooth or faintly faceted, in which it is flattened into multiple sides to form a prism-like geometry.[5]
Bardet et al. (2006) classified Tylosaurus species into two morphological groups based on marginal dentition. The North American 'proriger group' includes T. proriger and T. nepaeolicus and is characterized by teeth with smooth or faint facets, less prominent cutting edges, and a vein-like network of primitive striations extending to near the tip.[54] The group was originally defined as having slender teeth,[54] but subsequent research has since recognized that slenderness is an ontogenetic trait in T. proriger with robust teeth appearing in adult forms.[53] Though not formally classified within a group, the marginal teeth of T. saskatchwanensis shares a comparable morphology with T. proriger.[42] The second is the Euro-American 'ivoensis group' and consists of T. ivoensis, T. gaudryi, and T. pembinensis. Their teeth are robust with prominent cutting edges with striations on the lingual and occasionally buccal sides that do not reach the tooth's tip, and facets on the buccal side.[54] The facets are gentle in T. pembinensis,[36] while in T. ivoensis they are slightly concave.[49] The latter feature is also known as fluting.[55] Marginal teeth in T. gaudryi are virtually indistinguishable from those in T. ivoensis.[49] T. iembeensis was not placed within either group; no further description is known of its teeth other than having striations and no facets.[54] The distinction of an 'ivoensis group' is contentious. Caldwell et al. (2008) argued that T. pembinensis cannot be compared with T. ivoensis as the former's teeth are not fluted, and that T. ivoensis is more allied with the distinctively fluted teeth of Taniwhasaurus.[55] Jiménez-Huidobro and Caldwell (2019) listed the absence of marginal fluting as a diagnostic (taxon-identifying) trait that differentiates Tylosaurus from Taniwhasaurus.[6]
Pterygoid teeth are smaller and more recurved than marginal teeth.[46] The base of the former are nearly circular but divided into a front and back-facing side of near-equal surface area via a pair of faint buccal and lingual cutting edges. This is except in T. gaudryi, where the teeth are compressed at the lingual and labial sides and hold distinct cutting edges at the front and back sides.[49] Cutting edges are not serrated in all named species where it is known.[45][5] Past studies claimed to have identified serrations in T. bernardi; Jiménez-Huidobro and Caldwell (2016) found none.[45] The typical front-facing side is either smooth of faintly faceted, while the back-facing side is striated. Tooth build is robust in at least T. ivoensis.[49]
Cranium
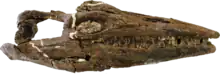
The most recognizable characteristic of Tylosaurus is the elongated conical rostrum that protrudes from its snout, from which the genus is named after. This is formed by the elongation of the front end of the premaxilla[44] and dentary.[42] Unlike typical mosasaurs, Tylosaurus did not have teeth up to the end of the snout nor on the bony prow that is the rostrum. This feature is not present at birth but rapidly developed during infancy[56] and is believed to have been primarily used for combat purposes such as ramming.[57][44][56] Once developed, the rostrum starts as an acutely-angled rounded shape in immature T. nepaeolicus and T. proriger, but takes on a broader rectangular form in mature individuals[56][38] and in T. saskatchewanensis,[42] T. bernardi,[45] and T. pembinensis.[36] The prow is supported by a broad and robust internarial bar (the extension of the premaxilla on top of the skull that held together the nasal and upper jaws), which provided effective shock absorption and stress transfer following a frontward collision.[44] Additional protective adaptations include a robust braincase that forms a sliding joint with the roof of the back of the skull, cushioning the brain from blunt-force trauma,[44] and in later species,[lower-alpha 1][36][45][42] an interlocking suture between the rostral portion of the premaxilla and front end of the maxilla to form a sinusoidal shape resembling a pair of buttresses, which acted as a reinforced strut.[44] The prow holds the terminal branches for the trigeminal nerves through randomly scattered foramina.[58] Exits are also lined along the maxilla right above the gum lines.[59]
The external nares, or body nostril openings, are another important diagnostic character. The premaxilla, maxilla, and frontal bones form its rims; unlike typical mosasaurs the prefrontal bones are not involved.[6] The nares open somewhere above the fourth maxillary tooth from the front end and extends until it touches the frontal; in T. proriger it opens directly above or slightly behind the tooth[lower-alpha 2], while in T. nepaeolicus it begins between the third and fourth maxillary teeth,[36] T. bernardi towards the end of the fourth tooth,[45] and T. pembinensis right above the same tooth.[36] Nare length relative to the skull varied between species. It is proportionally short in T. proriger at 20-26% of total skull length,[61][49] T. bernardi at around 24%,[36] and T. gaudryi at 25-27%,[49] while long in T. pembinensis at 28-31%.[36] The nasal bones occupied the back half of the external nares. They were either free-floating or lightly articulated to the internarial bar light sutures at the front ends.[44] The back ends reached close proximity to the frontal but not full contact.[61] The nasals' loose association with the rest of the skull may be why the bones are frequently lost and therefore exceedingly rare;[61] it is known in only two skulls of T. bernardi and T. sp. They came in free pairs that were independent of each other; this is unlike in living monitor lizards where the nasals are fused into one unit. Tylosaurus is the only mosasaur where free nasal pairs are clearly documented.[44] The only record of nasals in other mosasaurs[lower-alpha 3][44] is in the holotype of Plotosaurus, where one is missing,[64] so it unclear whether the paired trait is a plesiomorphy of just the tylosaurines or of mosasaurs broadly.[44] The external nares lead to the choanae (internal nares) at the bottom of the cranium, which provides passage from the nostrils to the throat.[65] In Tylosaurus, they are shaped like a compressed teardrop. The choanae are constrained in extent by the vomers and palatine bone to the back half of the maxilla.[66] Ahead of the choanae, each vomer holds a small groove that held the Jacobson's organ, which is involved in the tongue-based sense of smell. It begins opposite of the fourth maxillary tooth in Tylosaurus,[67] and also ends immediately past the fifth maxillary tooth in T. bernardi.[44] The exit point for the veins leading to sinuses inside the palatine occur right in front of the Jacobson's organ between the vomers and maxilla. This differs from living monitors, where the exit occurs behind the organ.[66]
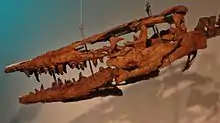
The frontal bone usually possesses a small sagittal crest along its midline. It is most prominent in T. proriger.[6][51] It is moderately developed in T. saskatchewanensis[42] and T. bernardi, and also extends into the premaxilla for the latter.[45] The frontal crest is present but poorly developed in most T. nepaeolicus skulls, and occasionally lost in some mature individuals.[38] The frontal overlaps parts of the prefrontals that do not form the orbits (eye sockets), and at its rear end, articulates with the parietal bone through a frontoparietal suture. The shape of the suture is plastic[6] and can be anywhere between straight to strongly M-shaped, which in at least T. nepaeolicus and T. proriger appear to be ontogenetic variations.[38] The position of the pineal eye on the parietal is likewise variable, either appearing close to the frontoparietal suture or directly overlapping with it.[45][51] The orbits are formed by the prefrontal, postorbitofrontal, and jugal bones. The prefrontals and postorbitofrontals overlap at the top of the orbits, preventing the frontal bone from contributing to the sockets' shape.[6] The jugal forms the bottom of the orbit; in Tylosaurus, it is L-shaped and has a distinctive serif-like extension at the lower back corner of the junction between the horizontal and vertical rami (arms) called the ventroposterior process.[lower-alpha 4][51] The vertical ramus is overlapped by the postorbitofrontal in most species,[42][36][51] and horizontal ramus by the maxilla in T. pembinensis.[36] In H. bernardi, the vertical ramus is not overlapped but joins with the postorbitofrontal by a suture, and is diagnostically much thicker than the horizontal ramus.[45]
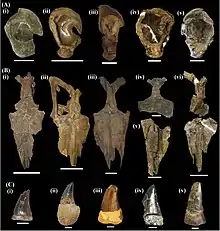
The quadrate bones are located at the back corners of the skull. They served as joints connecting the lower jaw to the cranium,[68] and carried the eardrum.[69] Several parts of the bone capture distinctive homologous variations[70] considered diagnostic up to the species level; some species can be differentiated based on the quadrate.[6] Ecology appears to be a key driver of quadrate morphology in squamates, and as a result may be a major factor for its variation between Tylosaurus species.[70] On the side facing away from median plane of the skull, the quadrate initially resembles a hook in immature T. nepaeolicus and T. proriger individuals, but in adult forms for both species[38] and in T. bernardi,[6][45] T. pembinensis,[36] and T. saskatchweanensis it takes on a robust oval-like shape.[42] The main body, known as the shaft, holds a broad concavity called the tympanic conch that takes up most of its extent in this view, which held the eardrum.[69] It is shallow in T. nepaeolicus,[70] T. proriger, and T. bernardi,[6] while deep and bowl-shaped in T. pembinensis[70] and T. saskatchewanensis.[6] The rim of the conch closer to the front of the skull is called the tympanic ala.[71] It is thin in T. nepaeolicus, T. proriger,[38] and T. bernardi,[45] also wide and flat in the former two,[38] thicker than T. bernardi in T. pembinensis,[45] and thick in T. saskatchewanensis.[42] Emerging from the top of the shaft is the suprastapedial process, which curves towards the backward direction of the skull into an incomplete loop and served as the attachment point for the depressor mandibulae muscles that open the lower jaw[36][72] through prominent insertion grooves on the top and side surfaces.[70] It is slender and proportionally long in immature T. nepaeolicus and T. proriger, giving their quadrates the hook-like appearance, but by adulthood is thick and reaches about half the length of the entire bone.[38] The process is of similar relative length in T. saskatchwanensis[42] but probably shorter in T. bernardi.[45] In T. pembinensis, it abruptly turns inward to face the skull's median plane at a 45° downward angle and therefore difficult to measure.[36] A similar deflection appears in some juvenile T. nepaeolicus quadrates.[51] Emerging from the lower back-facing rim of the quadrate is the infrastapedial process. Its shape changes during maturity in T. nepaeolicus and T. proriger. For the former, the process is absent in juveniles but appears as a small development in adults, while it is already present as a subtle point in juveniles and becomes a distinctively broad semicircle in adults for the latter.[38] It was probably poorly developed in T. bernardi.[45] In T. pembinensis[36] and T. saskatchewanensis,[42] the infrastapedial process is rounded and located high on the lower half of the rim; for the latter species the suprastapedial process almost touches it.[42] At the bottom of the shaft is the mandibular condyle, which is the joint connected to the lower jaw. It is rounded in all adult species where known,[70][45][42][38] but initially does not exist in infant T. nepaeolicus and T. proriger.[38] On the side facing towards the skull's median plane in at least T. nepaeolicus and T. pembinensis, there is a thick vertical ridge that lines the shaft's surface, which protrudes at the top of the quadrate like a pillar.[70]
Postcranial skeleton
Tylosaurus had 29 to 30 vertebrate between the skull and hip, 6 to 7 vertebrae in the hip, 33 to 34 vertebrae in the tail with chevrons, and a further 56 to 58 vertebrae making up the tip of the tail.[45]
Soft tissue
Skin and coloration
Fossil evidence of the skin of Tylosaurus in the form of scales has been described since the late 1870s. These scales were small and diamond-shaped and were arranged in oblique rows, comparable to that found in modern rattlesnakes and other related reptiles. However, the scales in the mosasaur were much smaller in proportion to the whole body.[73][74] An individual measuring 5 meters (16 ft) in total body length had dermal scales measuring 3.3 by 2.5 millimeters (0.130 in × 0.098 in),[75] and in each square inch (2.54 cm) of the mosasaur's underside an average of ninety scales were present.[73] Each scale was keeled in a form resembling that of a shark's denticles.[74] This probably helped reduce underwater drag[74] and reflection on the skin.[76]
Microscopic analysis of scales in a T. nepaeolicus specimen by Lindgren et al. (2014) detected high traces of the pigment eumelanin indicative of a dark coloration similar to the leatherback sea turtle in life. This may have been complemented with countershading, present in many aquatic animals, though the distribution of dark and light pigments in the species remains unknown. A dark-colored form would have provided several evolutionary advantages. Dark coloration increases absorption of heat, allowing the animal to maintain elevated body temperatures in colder environments. Possession of this trait during infancy would in turn facilitate fast growth rates. Unreflective dark coloring and countershading would have provided the mosasaur with increased camouflage. Additional speculative functions includes increased tolerance to solar ultraviolet radiation, strengthened integuments. The study remarked that certain melanism-coding genes are pleiotropic for increased aggression.[76]
Respiratory system
AMNH FR 221 preserves parts of the cartilaginous respiratory system. This includes parts of the larynx (voice box), trachea (windpipe), and bronchi (lung airways). They were however only briefly described in the preserved position by Osborn (1899). The larynx is poorly preserved; a piece of its cartilage first appears below just between the pterygoid and quadrate and extends to behind the latter. This connects to the trachea, which appears below the atlas vertebra but is not preserved afterwards. The respiratory tract reappears below the fifth rib as a pair of bronchi and extends to just behind the as-preserved coracoids where preservation is lost.[77] The pairing is suggestive of two functional lungs like modern limbed lizards but unlike snakes.[78] Similar branching is also found in Platecarpus[78] and putatively Mosasaurus, the only two other derived mosasaurs with their respiratory systems documented.[79] The bifurcation point for the Tylosaurus specimen is anywhere between the first and sixth cervical vertebrae.[lower-alpha 5][77] In Platecarpus, the bronchi probably diverged below the sixth cervical into near-parallel pairs,[80] while in Mosasaurus the organ is dislocated.[79] A bifurcation point's position ahead of the forelimbs would be unlike terrestrial lizards, whose point is within the chest region, but similar to the short trachea and parallel bronchi of whales.[78]
Classification
Taxonomy
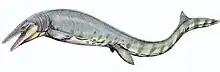
As a mosasaur, Tylosaurus is classified within the family Mosasauridae in the superfamily Mosasauroidea. The genus is the type genus of its own subfamily, the Tylosaurinae. Other members of this group include Taniwhasaurus and possibly Kaikaifilu, and the subfamily is defined by a shared feature of an elongated conical rostrum that does not bear teeth.[6] The closest relatives of the Tylosaurinae include the Plioplatecarpinae and the primitive subfamilies Tethysaurinae and Yaguarasaurinae; together they are members of one of three possible major lineages of mosasaurs (the others being the Mosasaurinae subfamily and Halisauromorpha group) that was first recognized in 1993. This clade was named the Russellosaurina by Polcyn and Bell in 2005.[81][82][83]
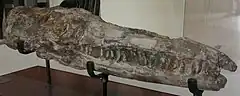
Tylosaurus was among the earliest derived mosasaurs. The oldest fossil attributable to the genus is of a premaxilla (TMM 40092-27) recovered from Middle Turonian deposits of the Arcadia Park Shale in Texas,[2] which is dated between 92.1-91.4 million years old based on correlations with index fossils.[1] Although formally referred to as Tylosaurinae incertae sedis during its first description, it was remarked to probably belong to T. kansasensis.[2] The specimen was later listed within the species in a 2020 reexamination.[38] A slightly younger specimen is of a skull (SGM-M1) of an indeterminate Tylosaurus species similar to T. kansasensis from the Ojinaga Formation in Chihuahua, Mexico,[3] dated around ~90 million years old at earliest.[1] A tooth from a Late Maastrichtian deposit in Nasiłów, Poland dating close to the Cretaceous–Paleogene boundary has been attributed to Hainosaurus sp.[4][8] With the incorporation of Hainosaurus as a synonym of Tylosaurus, this also makes the genus one of the last mosasaurs.[45][4] Currently, eight species of Tylosaurus are recognized by scientists as taxonomically valid. They are as follow: T. proriger, T. nepaeolicus, T. bernardi, T. gaudryi, T. ivoensis, T. iembeensis, T. pembinensis, and T. saskatchewanensis. The validity of two additional taxa remain unsettled; there is still debate whether T. kansasensis is synonymous with T. nepaeolicus, and T. borealis has yet to be described in a formal publication.[6][37]
Phylogeny and evolution
In 2020, Madzia and Cau performed a Bayesian analysis to better understand the evolutionary influence on early mosasaurs by contemporaneous pliosaurs and polycotylids by examining the rates of evolution in mosasauroids like Tylosaurus (specifically T. proriger, T. nepaeolicus, and T. bernardi). A Bayesian analysis in the study's implementation can approximate numerically-defined rates of morphological evolution and ages of divergence of clades. The Tylosaurinae was approximated to have diverged from the Plioplatecarpinae around 93 million years ago; the divergence was characterized by the highest rate of evolution among all mosasaurid lineages. This trend of rapid evolution coincided with the extinction of the pliosaurs and a decrease in polycotylid diversity. The study noted converging traits between Tylosaurus, pliosaurs, and some polycotylids in tooth morphology and body size. However, there was no evidence to suggest that Tylosaurus or its precursors evolved as a result of out-competing and/or driving to extinction the pliosaurs and polycotylids. Instead, Madiza and Cau proposed that Tylosaurus may have taken advantage of the extinction of the pliosaurs and decline of polycotylids to quickly fill the ecological void they left behind. The Bayesian analysis also approximated a divergence of T. nepaeolicus from the rest of the genus around 86.88 million years ago and a divergence between T. proriger and T. bernardi around 83.16 million years ago. The analysis also generated a paraphyletic status of the genus, approximating Taniwhasaurus to have diverged from Tylosaurus around 84.65 million years ago, but this result is not consistent with previous phylogenetic analyses.[84]
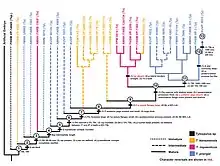
In the Western Interior Seaway, two species—T. nepaeolicus and T. proriger—represent a chronospecies, in which they make up a single lineage that continuously evolves without branching off in a process known as anagenesis. This is evident by how the two species do not stratigraphically overlap, are sister species, share minor and intermediate morphological differences such as a gradual change in the development of the quadrate bone, and lived in the same locations.[60][38] The means by which this lineage evolved has been hypothesized to be through one of two evolutionary mechanisms related to changes in ontogeny. First, Jiménez-Huidobro, Simões, and Caldwell proposed in 2016 that T. proriger evolved as a paedomorph of T. nepaeolicus, in which the descendant arose as a result of morphological changes through the retention of juvenile features of the ancestor in adulthood. This was based on the presence of a frontal crest and convex borders of the parietal bone of the skull shared in both juvenile T. nepaeolicus and all T. proriger but lost in adult T. nepaeolicus.[51][38] However, an ontogenetic study by Zietlow (2020) found that it was unclear whether this observation was a result of paedomorphosis, although this uncertainty may have been due that the sample size of mature T. nepaeolicus was too low to determine statistical significance. Second, the same study proposed an alternative hypothesis of peramorphosis, in which T. proriger evolved by developing traits found in mature T. nepaeolicus during immaturity. Based on results from a cladistical ontogram developed using data from 74 Tylosaurus specimens, the study identified a multitude of traits that were present in all T. proriger and mature T. nepaeolicus but absent in juvenile T. nepaeolicus: the skull size and depth are large, the length of the elongated rostrum exceeds 5% of the total skull length, the quadrate suprastapedial processes are thick, the overall quadrate shape converges, and the posteroventral process is fan-like.[38]
The following cladogram is modified from a phylogenetic analysis by Jiménez-Huidobro & Caldwell (2019) using Tylosaurus species with sufficiently known material to model accurate relationships; T. gaudryi, T. ivoensis, and T. iembeensis were too poorly understood to be added into the analysis.[6]
| |||||||||||||||||||||||||||||||||||||||||||||||||||||||||||||||||||||||||||||||||||||||||||||||||||||||||||||||||||||||||||||||||||||
Paleobiology
Growth
Konishi and colleagues in 2018 assigned specimen FHSM VP-14845, a small juvenile with an estimated skull length of 30 centimeters (12 in), to Tylosaurus based on the proportions of the braincase and the arrangement of the teeth in the snout and on the palate. However, the specimen lacks the characteristic snout projection of other Tylosaurus, which is present in juveniles of T. nepaeolicus and T. proriger with skull lengths of 40–60 cm (16–24 in). This suggests that Tylosaurus acquired the snout projection rapidly at an early stage in life, and also suggests that it did not develop due to sexual selection. Konishi and colleagues suggested a function in ramming prey, as employed by the modern orca.[56]
Metabolism
Nearly all squamates are characterized by their cold-blooded ectothermic metabolism, but mosasaurs like Tylosaurus are unique in that they were likely endothermic, or warm-blooded.[85] The only other known lizard with such a trait is the Argentine black and white tegu, though only partially.[86] Endothermy in Tylosaurus was demonstrated in a 2016 study by Harrell, Pérez‐Huerta, and Suarez by examining δ18O isotopes in Tylosaurus bones. δ18O levels can be used to calculate the internal body temperature of animals, and by comparing such calculated temperatures between coexisting cold-blooded and warm-blooded animals, the type of metabolism can be inferred. The study used the body temperatures of the cold-blooded fish Enchodus and sea turtle Toxochelys (correlated with ocean temperatures) and warm-blooded seabird Ichthyornis from the Mooreville Chalk as a proxy. Analyzing the isotope levels of eleven Tylosaurus specimens an average internal body temperature of 34.3 °C (93.7 °F) was calculated. This was much higher than the body temperature of Enchodus and Toxochelys (28.3 °C (82.9 °F) and 27.2 °C (81.0 °F) respectively) and similar to that of Ichthyornis (38.6 °C (101.5 °F)). Harrell, Pérez‐Huerta, and Suarez also calculated the body temperatures of Platecarpus and Clidastes with similar numbers, 36.3 °C (97.3 °F) and 33.1 °C (91.6 °F) respectively. The fact that the other mosasaurs were much smaller in size than Tylosaurus and yet maintained similar body temperatures made it unlikely that Tylosaurus's body temperature was the result of another metabolic type like gigantothermy.[lower-alpha 6] Endothermy would have provided several advantages to Tylosaurus such as increased stamina for foraging larger areas and pursuing prey, the ability to access colder waters, and better adaptation to withstand the gradual cooling of global temperatures during the Late Cretaceous.[85]
Mobility
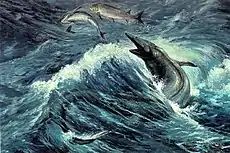
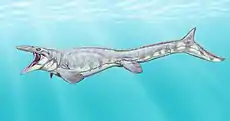
Scientists previously interpreted Tylosaurus as an anguilliform swimmer that moved by undulating its entire body like a snake due to its close relationship with the animal. However, it is now understood that Tylosaurus actually used carangiform locomotion, meaning that the upper body was less flexible and movement was largely concentrated at the tail like in mackerels. A BS thesis by Jesse Carpenter published in 2017 examined the vertebral mobility of T. proriger spinal columns and found that the dorsal vertebrae were relatively rigid but the cervical, pygal, and caudal vertebrae were more liberal in movement, indicating flexibility in the neck, hip, and tail regions. This contrasted with more derived mosasaurs like Plotosaurus, whose vertebral column was stiff up to the hip. Interestingly, an examination of a juvenile T. proriger found that its cervical and dorsal vertebrae were much stiffer than those in adult specimens. This may have been an evolutionary adaptation among young individuals; a more rigid tail-based locomotion is associated with faster speed, and this would allow vulnerable juveniles to better escape predators or catch prey. Older individuals would see their spine grow in flexibility as predator evasion becomes less important for survival.[88]
Tylosaurus likely specialized as an ambush predator. It was lightweight for a mosasaur of its size, having a morphological build designed to vastly reduce body mass and density. Its pectoral and pelvic girdles and paddles, which are associated with weight, are proportionally small. Its bones were highly cancellous and were likely filled with fat cells in life, which also increased buoyancy. It is unlikely that the latter trait was evolved in response to increasing body size as the similarly-sized Mosasaurus hoffmannii lacked highly cancellous bone. These traits allowed Tylosaurus to be more conservative in its energy requirements, which is useful when traveling between ambush sites over large distances or through stealth. In addition, a reduced body density likely helped Tylosaurus to rapidly accelerate during an attack, assisted with the long and powerful tail of the mosasaur.[44]
A 1988 study by Judith Massare attempted to calculate the sustained swimming speed, the speed at which the animal moves without tiring, of Tylosaurus through a series of mathematical models incorporating hydrodynamic characteristics and estimations of locomotive efficiency and metabolic costs. Using two T. proriger specimens, one 6.46 meters (21.2 ft) long and the other 6.32 meters (20.7 ft), she calculated a consistent average maximum sustained swimming speed of 2.32 m/s (5.2 mph). However, when testing whether the models represented an accurate framework, they were found to exaggerated. This was primarily because the variables accounting for drag may have been underestimated; estimation of drag coefficients for an extinct species can be difficult as it requires a hypothetical reconstruction of the morphological dimensions of the animal. Massare predicted that the actual sustained swimming speed of Tylosaurus was somewhere near half the calculated speed.[89]
Feeding
.svg.png.webp)
One of the largest marine carnivores of its time, Tylosaurus was an apex predator that exploited the wide variety of marine fauna in its ecosystem. Stomach contents are well documented in the genus, which includes other mosasaurs, plesiosaurs, turtles, birds, bony fish, and sharks.[90] Additional evidence from bite marks suggests the animal also preyed on giant squid[91] and ammonites.[92]
The enormous and varied appetite of Tylosaurus can be demonstrated in a 1987 find that identified fossils of a mosasaur measuring 2 meters (6.6 ft) or longer, the diving bird Hesperornis, a Bananogmius fish, and possibly a shark all within the stomach of a single T. proriger skeleton (SDSM 10439) recovered from the Pierre Shale of South Dakota.[lower-alpha 7][44][90][95] Other records of stomach contents include a sea turtle in a T. bernardi-like species,[lower-alpha 8][90] a 2.5 meters (8.2 ft) long Dolichorhynchops in another (8.8 metres (29 ft) long) T. proriger,[29] partially digested bones and scales of a Cimolichthys in a third T. proriger,[93] partially digested vertebrae of a Clidastes in a fourth T. proriger, remains of three Platecarpus individuals in a T. nepaeolicus,[28] and Plioplatecarpus bones in a T. saskatchewanensis.[33][96] Puncture marks on fossils of ammonites,[92] the carapace of a Protostega,[97] and the gladius of an Enchoteuthis have been attributed to Tylosaurus.[91]
Pasch and May (2001) reported bite marks from a dinosaur skeleton known as the Talkeetna Mountains Hadrosaur, which was found in marine strata of the Turonian-age Matanuska Formation in Alaska. The features of these marks were found to closely match that of the teeth of T. proriger. Because the fossil's locality was of marine deposits, the study reasoned that the dinosaur must have drifted offshore as a bloat-and-float carcass that was subsequently scavenged by the mosasaur. It was unlikely that the marks were a result of predation, as that would have led to a puncture, preventing the buildup of the bloating gases that allowed the corpse to drift out to sea in the first place.[98] Garvey (2020) criticized the lack of conclusive evidence to support this hypothesis and ruled out T. proriger as a possible culprit, given that the species did not appear until the Santonian and is exclusive to the Western Interior Seaway.[37] However, close relatives did maintain a presence nearby, evidenced by fragmentary fossils of an indeterminate tylosaurine from Turonian deposits in the Russian Chukotsky District.[99]
Social behavior
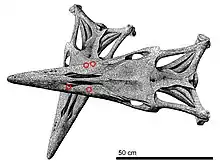
The behavior of Tylosaurus towards each other may have been mostly aggressive, evidenced by fossils with injuries inflicted by another of their own kind. Such remains were frequently reported by fossil hunters during the late 19th and early 20th centuries, but few examples reside as specimens in scientific collections. Many of these fossils consist of healed bite marks and wounds that are concentrated around or near the head region, implying that there were the result of non-lethal interaction, but the motives of such contact remain speculative. In 1993, Rothschild and Martin noted that some modern lizards affectionately bite their mate's head during courtship, which can sometimes result in injuries. Alternatively, they also observed that some males lizards also employ head-biting as territorial behavior against rivals in a show of dominance by grappling the head to turn over the other on its back. It is possible that Tylosaurus behaved in similar ways.[28]
Lingham-Soliar (1992) noted suggestions that use of the combat-oriented elongated rostrum of Tylosaurus was not exclusive to hunting and that it may have also been applied in sexual behavior through battles over female mates between males.[44] However, he observed the elongated rostrum was invariably present in all individuals regardless of sex,[44] and subsequent studies by Konishi et al. (2018) and Zietlow (2020) confirmed this pattern.[56][38] This would imply that sexual selection was not a driver in its evolution and instead refined through sex-independent means.[56]
At least one fatal instance of intraspecific combat among Tylosaurus is documented. This can be seen in the T. kansasensis holotype FHSM VP-2295, representing a 5 meters (16 ft) long animal, which possesses numerous injuries that indicate it was killed by a larger Tylosaurus. The skull roof and surrounding areas exhibit signs of trauma in the form of four massive gouges, and the dentary contains at least seven puncture wounds and gouges. These pathologies are unequivocally characteristic of bite marks from a larger Tylosaurus that measured around 7 meters (23 ft) in length. The largest of the marks are about 4 centimeters (1.6 in) in length, matching the size of large mosasaur teeth, and they are positioned along two lines that converge close to 30°, matching the angle that each jaw converges towards in a mosasaur skull. In addition, FHSM VP-2295 suffered damage to the sclerotic ring, a detached quadrate, and an articulation of the cervical vertebrae at an unnatural angle of 40° to the skull's long axis. The pattern of preservation makes it unlikely that the condition of the vertebrae was a result of disturbances by scavengers and instead indicates damage caused by a violently twisted neck during life. In a reconstructed scenario, the larger Tylosaurus would have first attacked at an angle slightly below the left side of the victim's head. This impact would cause the victim's skull to roll to the right side, allowing the aggressor to sink its teeth into the skull roof and right lower jaw, the latter crushing the jawbone and causing further breaks of nearby bones such as the pterygoid, a break in the right eye, and the twisting of the jawbone outwards. This would subsequently cause the quadrate to detach from its position and the spinal cord to twist and sever at the skull's base, leading to a swift death.[28]
Paleopathology
Examining 12 North American Tylosaurus skeletons and one T. bernardi skeleton, Rothschild and Martin (2005) identified evidence of avascular necrosis in every individual. For aquatic animals, this condition is often a result of decompression illness, which is caused when bone-damaging nitrogen bubbles build up in inhaled air that is decompressed either by frequent deep-diving trips or by intervals of repetitive diving and short breathing. The studied mosasaurs likely gained avascular necrosis through such behaviors, and given its invariable presence in Tylosaurus it is likely that deep or repetitive diving was a general behavioral trait of the genus. The study observed that between 3-15% of vertebrae in the spinal column of North American Tylosaurus and 16% of vertebrae in T. bernardi were affected by avascular necrosis.[100] Carlsen (2017) posited that Tylosaurus gained avascular necrosis because it lacked the necessary adaptations for deep or repetitive diving, although noted that the genus had well-developed eardrums that could protect themselves from rapid changes in pressure[101]
Unnatural fusion of some vertebrae in the tail has been reported in some Tylosaurus skeletons. A variation of these fusions may concentrate near the end of the tail to form a single mass of multiple fused vertebrae called a "club tail." Rothschild and Everhart (2015) surveyed 23 North American Tylosaurus skeletons and one T. bernardi skeleton and found that five of the North American skeletons exhibited fused tail vertebrae. The condition was not found in T. bernardi, but this does not rule out its presence due to the low sample size. Vertebral fusion occurs when the bones remodel themselves after damage from trauma or disease. However, the cause of such events can vary between individuals and/or remain hypothetical. One juvenile specimen with the club tail condition was found with a shark tooth embedded in the fusion, which confirms that at least some cases were caused by infections inflicted by predator attacks. The majority of vertebral fusion cases in Tylosaurus were caused by bone infections, but some cases may have alternatively been caused by any type of joint disease such as arthritis. However, evidence of joint disease was rare in Tylosaurus when compared to mosasaurs such as Plioplatecarpus and Clidastes.[102] Similar amassing of remodeled bone is also documented in bone fractures in other body parts. One T. kansasensis specimen possesses two fractured ribs that fully healed. Another T. proriger skull shows a fractured snout, probably caused by ramming into a hard object such as a rock. Presence of some healing indicates that the individual survived for some extended time before death. The injury in a snout region containing many nerve endings would have inflicted extreme pain.[103]
Notes
- ↑ Inconsistent in T. proriger.[38]
- ↑ Behind the fifth tooth in the holotype.[60]
- ↑ Nasals were initially identified in the holotype of Eonatator,[62] but later found to be a plaster restoration.[63]
- ↑ In one juvenile T. proriger specimen, it appears at the bottom of the vertical ramus instead.[51]
- ↑ Latter corresponds to the fifth rib in Osborn (1899).[77]
- ↑ The 2018 MS thesis of Cyrus Green disputes the notion that Clidastes was an endotherm based on the skeletochronology of the genus, finding that its growth rates were too low to be endothermic and instead similar to ectotherms. The dissertation argued that the high body temperatures calculated by Harrell et al. (2016) were a result of gigantothermy. However, only four specimens were studied, and Clidastes is considered a basal mosasaur.[87]
- ↑ Identification of the mosasaur and shark vary. Scientists have identified the mosasaur as either a Platecarpus,[28] Clidastes,[93] or Latoplatecarpus.[90] The shark is either interpreted as a Cretalamna,[93] a sand shark,[94] or of uncertain identity.[90]
- ↑ Usually identified as Hainosaurus sp.;[90] Lingham-Soliar (1992) identifies the species as T. bernardi.[44]
References
- 1 2 3 Ogg, J.G.; Hinnov, L.A. (2012), "Cretaceous", in Gradstein, F. M.; Ogg, J. G.; Schmitz, M. D.; Ogg, G. M. (eds.), The Geologic Time Scale, Oxford: Elsevier, pp. 793–853, doi:10.1016/B978-0-444-59425-9.00027-5, ISBN 978-0-444-59425-9, S2CID 127523816
- 1 2 3 Polycn, M.J.; Bell Jr., G.L.; Shimada, K.; Everhart, M.J. (2008). "The oldest North American mosasaurs (Squamata: Mosasauridae) from the Turonian (Upper Cretaceous) of Kansas and Texas with comments on the radiation of major mosasaur clades". Proceedings of the Second Mosasaur Meeting: 137–155.
- 1 2 3 Abelaid Loera Flores (2013). "Occurrence of a tylosaurine mosasaur (Mosasauridae; Russellosaurina) from the Turonian of Chihuahua State, Mexico" (PDF). Boletín de la Sociedad Geológica Mexicana. 65 (1): 99–107. doi:10.18268/BSGM2013v65n1a8.
- 1 2 3 J.W.M. Jagt; J. Lindgren; M. Machalski; A. Radwański (2005). "New records of the tylosaurine mosasaur Hainosaurus from the Campanian-Maastrichtian (Late Cretaceous) of central Poland". Netherlands Journal of Geosciences. 84 (Special Issue 3): 303–306. Bibcode:2005NJGeo..84..303J. doi:10.1017/S0016774600021077.
- 1 2 3 4 J.J. Hornung; M. Reich (2015). "Tylosaurine mosasaurs (Squamata) from the Late Cretaceous of northern Germany". Netherlands Journal of Geosciences. 94 (1): 55–71. Bibcode:2015NJGeo..94...55H. doi:10.1017/njg.2014.31. S2CID 129384273.
- 1 2 3 4 5 6 7 8 9 10 11 12 13 14 15 16 Paulina Jiménez-Huidobro; Michael W. Caldwell (2019). "A New Hypothesis of the Phylogenetic Relationships of the Tylosaurinae (Squamata: Mosasauroidea)". Frontiers in Earth Science. 7 (47): 47. Bibcode:2019FrEaS...7...47J. doi:10.3389/feart.2019.00047. S2CID 85513442.
- ↑ Louis L. Jacobs; Octávio Mateus; Michael J. Polcyn; Anne S. Schulp; Miguel Telles Antunes; Maria Luísa Morais; Tatiana da Silva Tavares (2006). "The occurrence and geological setting of Cretaceous dinosaurs, mosasaurs, plesiosaurs, and turtles from Angola" (PDF). Paleontological Society of Korea. 22 (1): 91–110.
- 1 2 Norbert Keutgen; Zbigniew Remin; John W.M. Jagt (2017). "The late Maastrichtian Belemnella kazimiroviensis group (Cephalopoda, Coleoidea) in the Middle Vistula valley (Poland) and the Maastricht area (the Netherlands, Belgium) – taxonomy and palaeobiological implications". Palaeontologia Electronica. 20.2.38A: 1–29. doi:10.26879/671.
- 1 2 3 4 Everhart 2017, p. 206.
- 1 2 3 Ellis 2003, p. 207.
- 1 2 Cope, E. D. (1869). "[Remarks on Holops brevispinus, Ornithotarsus immanis, and Macrosaurus proriger]". Proceedings of the Academy of Natural Sciences of Philadelphia. 1869: 123. ISSN 0097-3157.
- ↑ Everhart, M.J. (2005). "Macrosaurus proriger". Oceans of Kansas. Archived from the original on March 14, 2016.
- 1 2 Cope, E. D. (1870). "Synopsis of the extinct Batrachia, Reptilia and Aves of North America". Transactions of the American Philosophical Society. 14 (1): 1–252. doi:10.5962/bhl.title.60499.
- ↑ Everhart 2017, p. 207.
- ↑ "Sebastes proriger, Redstripe rockfish". FishBase. Archived from the original on October 19, 2015.
- 1 2 Marsh, O.C. (1872). "On the structure of the skull and limbs in mosasaurid reptiles, with descriptions of new genera and species". American Journal of Science. Series 3. 3 (18): 448–464.
- ↑ Russell 1967, p. 174.
- 1 2 Everhart, M.J. (2000). "Tylosaurus proriger". Oceans of Kansas. Archived from the original on October 16, 2012.
- ↑ Russell 1967, p. 173.
- ↑ Marsh, O.C. (1872). "Note on Rhinosaurus". American Journal of Science. 4 (20): 147.
- 1 2 3 4 5 Everhart, M.J. (2002). "New Data on Cranial Measurements and Body Length of the Mosasaur, Tylosaurus nepaeolicus (Squamata; Mosasauridae), from the Niobrara Formation of Western Kansas". Transactions of the Kansas Academy of Science. 105 (1–2): 33–43. doi:10.1660/0022-8443(2002)105[0033:NDOCMA]2.0.CO;2. S2CID 86314572.
- ↑ Leidy 1873, p. 271-276.
- ↑ Merriam, J.C. (1894). "Über die Pythonomorphen der Kansas-Kreide". Palaeontographica (in German). 41: 1–39.
- ↑ Everhart, M.J.; Jagt, J.W.M.; Mulder, E.W.A.; Schulp, A.S. (2016), Mosasaurs–how large did they really get? 5th triennial Mosasaur meeting—a global perspective on Mesozoic marine amniotes, Uppsala, 16–20 May 2016, Program and Abstracts, Museum of Evolution. Uppsala. Uppsala University. pp. 8-10
- ↑ Everhart 2017, p. 214.
- ↑ Everhart 2017, p. 192.
- ↑ Everhart, M.J. (2005). "Rapid evolution, diversification and distribution of mosasaurs (Reptilia; Squamata) prior to the KT Boundary". Tate 2005 11th Annual Symposium in Paleontology and Geology.
- 1 2 3 4 5 Everhart, M.J. (2008). "A bitten skull of Tylosaurus kansasensis (Squamata: Mosasauridae) and a review of mosasaur-on-mosasaur pathology in the fossil record". Transactions of the Kansas Academy of Science. 111 (3/4): 251–262. doi:10.1660/0022-8443-111.3.251. S2CID 85647383.
- 1 2 Everhart, M.J. (2004). "Plesiosaurs as the food of mosasaurs; new data on the stomach contents of a Tylosaurus proriger (Squamata; Mosasauridae) from the Niobrara Formation of western Kansas". The Mosasaur. 7: 41–46.
- ↑ Everhart 2017, p. 213.
- ↑ Everhart 2017, p. 215.
- 1 2 Lindgren, J. (2005). "The first record of Hainosaurus (Reptilia: Mosasauridae) from Sweden". Journal of Paleontology. 79 (6): 1157–1165. doi:10.1666/0022-3360(2005)079[1157:tfrohr]2.0.co;2.
- 1 2 "Omācīw". Royal Saskatchewan Museum. Archived from the original on October 1, 2020.
- ↑ "Largest mosasaur on display". Guinness World Records. Retrieved June 3, 2020.
- ↑ CBC News (August 27, 2008). "Manitoba dig uncovers 80-million-year-old sea creature". CBC. Manitoba. Archived from the original on June 5, 2018.
- 1 2 3 4 5 6 7 8 9 10 11 12 13 14 15 16 17 18 Bullard, T.S.; Caldwell, M.W. (2010). "Redescription and rediagnosis of the tylosaurine mosasaur Hainosaurus pembinensis Nicholls, 1988, as Tylosaurus pembinensis (Nicholls, 1988)". Journal of Vertebrate Paleontology. 30 (2): 416–426. Bibcode:2010JVPal..30..416B. doi:10.1080/02724631003621870. S2CID 86297189.
- 1 2 3 4 5 Garvey, S.T. (2020). A new high-latitude Tylosaurus (Squamata, Mosasauridae) from Canada with unique dentition (MS). University of Alberta. Archived from the original on July 23, 2020.
- 1 2 3 4 5 6 7 8 9 10 11 12 13 14 15 16 17 18 19 20 Zietlow, A.R. (2020). "Craniofacial ontogeny in Tylosaurinae". PeerJ. 8: e10145. doi:10.7717/peerj.10145. PMC 7583613. PMID 33150074.
- ↑ Christiansen, P.; Bonde, M. (2002). "A new species of gigantic mosasaur from the Late Cretaceous of Israel". Journal of Vertebrate Paleontology. 22 (3): 629–644. doi:10.1671/0272-4634(2002)022[0629:ANSOGM]2.0.CO;2. S2CID 86139978.
- ↑ Russell 1967, p. 14.
- ↑ Nicholls, E.L. (1988). "The first record of the mosasaur Hainosaurus (Reptilia: Lacertilia) from North America". Canadian Journal of Earth Sciences. 25 (10): 1564–1570. Bibcode:1988CaJES..25.1564N. doi:10.1139/e88-149.
- 1 2 3 4 5 6 7 8 9 10 11 12 13 14 15 Jiménez-Huidobro, P.; Caldwell, M.W.; Paparella, I.; Bullard, T.S. (2018). "A new species of tylosaurine mosasaur from the upper Campanian Bearpaw Formation of Saskatchewan, Canada". Journal of Systematic Palaeontology. 17 (10): 1–16. doi:10.1080/14772019.2018.1471744. S2CID 90533033.
- ↑ Russell 1967, p. 50.
- 1 2 3 4 5 6 7 8 9 10 11 12 13 14 15 16 Lingham-Soliar, T. (1992). "The Tylosaurine Mosasaurs (Reptilia, Mosasauridae) from the Upper Cretaceous of Europe and Africa". Bulletin de l'Institut Royal des Sciences Naturelles de Belgique, Sciences de la Terre. 62: 171–194. Archived from the original on November 12, 2021. Retrieved December 24, 2020.
- 1 2 3 4 5 6 7 8 9 10 11 12 13 14 15 16 17 18 19 Jiménez-Huidobro, P.; Caldwell, M.W. (2016). "Reassessment and reassignment of the early Maastrichtian mosasaur Hainosaurus bernardi Dollo, 1885, to Tylosaurus Marsh, 1872". Journal of Vertebrate Paleontology. 36 (3): e1096275. Bibcode:2016JVPal..36E6275J. doi:10.1080/02724634.2016.1096275. S2CID 87315531.
- 1 2 Russell 1967, p. 43.
- ↑ Lingham-Soliar, T. (1995). "Anatomy and functional morphology of the largest marine reptile known, Mosasaurus hoffmanni (Mosasauridae, Reptilia) from the Upper Cretaceous, Upper Maastrichtian of The Netherlands". Philosophical Transactions of the Royal Society B. 347 (1320): 155–180. Bibcode:1995RSPTB.347..155L. doi:10.1098/rstb.1995.0019. JSTOR 55929. S2CID 85767257. Archived from the original on October 26, 2019.
- 1 2 Russell 1967, p. 57.
- 1 2 3 4 5 6 7 8 9 10 11 Lindgren, J.; Siverson, M. (2002). "Tylosaurus ivoensis: a giant mosasaur from the early Campanian of Sweden". Earth and Environmental Science Transactions of the Royal Society of Edinburgh. 93 (1): 73–93. doi:10.1017/s026359330000033x. S2CID 131368986.
- ↑ Ross, M.R. (2009). "Charting the Late Cretaceous seas: mosasaur richness and morphological diversification". Journal of Vertebrate Paleontology. 29 (2): 409–416. Bibcode:2009JVPal..29..409R. doi:10.1671/039.029.0212. S2CID 55363105.
- 1 2 3 4 5 6 7 8 9 10 11 Jiménez-Huidobro, P.; Simões, T.R.; Caldwell, M.W. (2016). "Re-characterization of Tylosaurus nepaeolicus (Cope, 1874) and Tylosaurus kansasensis Everhart, 2005: Ontogeny or sympatry?". Cretaceous Research. 65: 68–81. Bibcode:2016CrRes..65...68J. doi:10.1016/j.cretres.2016.04.008.
- ↑ Stewart, R.F.; Mallon, J. (2018). "Allometric growth in the skull of Tylosaurus proriger (Squamata: Mosasauridae) and its taxonomic implications". Vertebrate Anatomy Morphology Palaeontology. 66: 75–90. doi:10.18435/vamp29339.
- 1 2 Konishi, T.; Caldwell, M. (2007). "Ecological and evolutionary implications of ontogenetic changes in the marginal dentition of Tylosaurus proriger (Squamata: Mosasauridae)" (PDF). Journal of Vertebrate Paleontology. 27 (3, Supplement): 101A. Archived from the original (PDF) on November 13, 2021.
- 1 2 3 4 Bardet, N.; Pereda Suberbiola, X.; COrral, J.C. (2006). "A Tylosaurine Mosasauridae (Squamata) from the Late Cretaceous of the Basque-Cantabrian Region". Estudios Geológicos. 62 (1): 213–218. doi:10.3989/egeol.0662121.
- 1 2 Caldwell, M.W.; Konishi, T.; Obata, I.; Muramoto, K. (2008). "A new species of Taniwhasaurus (Mosasauridae, Tylosaurinae) from the upper Santonian-lower Campanian (Upper Cretaceous) of Hokkaido, Japan". Journal of Vertebrate Paleontology. 28 (2): 339–348. doi:10.1671/0272-4634(2008)28[339:ansotm]2.0.co;2. S2CID 129446036.
- 1 2 3 4 5 6 Konishi, T.; Jiménez-Huidobro, P.; Caldwell, M.W. (2018). "The Smallest-Known Neonate Individual of Tylosaurus (Mosasauridae, Tylosaurinae) Sheds New Light on the Tylosaurine Rostrum and Heterochrony". Journal of Vertebrate Paleontology. 38 (5): 1–11. Bibcode:2018JVPal..38E0835K. doi:10.1080/02724634.2018.1510835. S2CID 91852673.
- ↑ Russell 1967, p. 69.
- ↑ Russell 1967, p. 16.
- ↑ Russell 1967, p. 17.
- 1 2 Everhart, M.J. (2005). "Tylosaurus kansasensis, a new species of tylosaurine (Squamata, Mosasauridae) from the Niobrara Chalk of western Kansas, USA". Netherlands Journal of Geosciences. 84 (3): 231–240. Bibcode:2005NJGeo..84..231E. doi:10.1017/S0016774600021016.
- 1 2 3 Russell 1967, p. 18.
- ↑ Wiman, C. (1920). "Some Reptiles from the Niobrara Group in Kansas" (PDF). University of Upsala, Uppsala. 18: 9–18.
- ↑ Bardet, N.; Suberbiola, X.P. (2001). "The basal mosasaurid Halisaurus sternbergii from the Late Cretaceous of Kansas (North America): a review of the Uppsala type specimen". Comptes Rendus de l'Académie des Sciences - Series IIA - Earth and Planetary Science. 332 (6): 395–402. Bibcode:2001CRASE.332..395B. doi:10.1016/S1251-8050(00)01486-5.
- ↑ Camp, C.L. (1942). "California mosasaurs". Memoirs of the University of California. 13: 1–68.
- ↑ Schulp, A.S.; Mulder, E.W.A.; Schwenk, K. (2005). "Did mosasaurs have forked tongues?". Netherlands Journal of Geosciences. 84 (3): 359–371. Bibcode:2005NJGeo..84..359S. doi:10.1017/S0016774600021144.
- 1 2 Russell 1967, p. 25.
- ↑ Russell 1967, p. 26.
- ↑ Russell 1967, p. 59.
- 1 2 Fernández, M.S.; Talevi, M. (2015). "An halisaurine (Squamata: Mosasauridae) from the Late Cretaceous of Patagonia, with a preserved tympanic disc: Insights into the mosasaur middle ear". Comptes Rendus Palevol. 14 (6–7): 483–493. Bibcode:2015CRPal..14..483F. doi:10.1016/j.crpv.2015.05.005. hdl:11336/54768.
- 1 2 3 4 5 6 7 Palci, A.; Konishi, T.; Caldwell, M.W. (2020). "A comprehensive review of the morphological diversity of the quadrate bone in mosasauroids (Squamata: Mosasauroidea), with comments on the homology of the infrastapedial process". Journal of Vertebrate Paleontology. 40 (6): e1879101. Bibcode:2020JVPal..40E9101P. doi:10.1080/02724634.2021.1879101. S2CID 233697990.
- ↑ Russell 1967, p. 47.
- ↑ Russell 1967, p. 61.
- 1 2 Snow, F.H. (1878). "On the dermal covering of a mosasauroid reptile". Transactions of the Kansas Academy of Science. 6: 54–58.
- 1 2 3 Caldwell, M.W.; Sasso, C.D. (2004). "Soft-tissue preservation in a 95 million year old marine lizard: form, function, and aquatic adaptation". Journal of Vertebrate Paleontology. 24 (4): 980–985. doi:10.1671/0272-4634(2004)024[0980:spiamy]2.0.co;2. S2CID 85605603.
- ↑ Lindgren, J.; Everhart, M.J.; Caldwell, M.W. (2011). "Three-Dimensionally Preserved Integument Reveals Hydrodynamic Adaptations in the Extinct Marine Lizard Ectenosaurus (Reptilia, Mosasauridae)". PLOS ONE. 6 (11): e27343. Bibcode:2011PLoSO...627343L. doi:10.1371/journal.pone.0027343. PMC 3217950. PMID 22110629.
- 1 2 Lindgren, J.; Sjövall, P.; Carney, R.M.; Uvdal, P.; Gren, J.A.; Dyke, G.; Schultz, B.P.; Shawkey, M. D.; Barnes, K. R. (2014). "Skin pigmentation provides evidence of convergent melanism in extinct marine reptiles". Nature. 506 (7489): 484–488. Bibcode:2014Natur.506..484L. doi:10.1038/nature12899. PMID 24402224. S2CID 4468035.
- 1 2 3 Osborn, H.F. (1899). "A complete mosasaur skeleton, osseous and cartilaginous". Memoirs of the American Museum of Natural History. 1 (4): 167–188. Bibcode:1899Sci....10..919O. doi:10.1126/science.10.260.919. hdl:2246/5737. PMID 17837338.
- 1 2 3 Lindgren, J.; Caldwell, M.W.; Konishi, T.; Chiappe, L.M. (2010). "Convergent Evolution in Aquatic Tetrapods: Insights from an Exceptional Fossil Mosasaur". PLOS ONE. 5 (8): e11998. Bibcode:2010PLoSO...511998L. doi:10.1371/journal.pone.0011998. PMC 2918493. PMID 20711249.
- 1 2 Konishi, T.; Newbrey, M.G.; Caldwell, M.W. (2014). "A small, exquisitely preserved specimen of Mosasaurus missouriensis (Squamata, Mosasauridae) from the upper Campanian of the Bearpaw Formation, western Canada, and the first stomach contents for the genus". Journal of Vertebrate Paleontology. 34 (4): 802–819. Bibcode:2014JVPal..34..802K. doi:10.1080/02724634.2014.838573. JSTOR 24523386. S2CID 86325001.
- ↑ Konishi, T.; Lindgren, J.; Caldwell, M.W.; Chiappe, L. (2012). "Platecarpus tympaniticus (Squamata, Mosasauridae): osteology of an exceptionally preserved specimen and its insights into the acquisition of a streamlined body shape in mosasaurs". Journal of Vertebrate Paleontology. 6 (12): 1313–1327. Bibcode:2012JVPal..32.1313K. doi:10.1080/02724634.2012.699811. S2CID 84208756.
- ↑ Bell, G.L. Jr.; Polcyn, M.J. (2005). "Dallasaurus turneri, a new primitive mosasauroid from the Middle Turonian of Texas and comments on the phylogeny of the Mosasauridae (Squamata)". Netherlands Journal of Geoscience. 84 (3): 177–194. Bibcode:2005NJGeo..84..177B. doi:10.1017/S0016774600020965.
- ↑ Caldwell, M.W. (2012). "A challenge to categories: "What, if anything, is a mosasaur?"". Bulletin de la Société Géologique de France. 183 (1): 17–34. doi:10.2113/gssgfbull.183.1.7.
- ↑ Madzia, D.; Cau, A. (2017). "Inferring 'weak spots' in phylogenetic trees: application to mosasauroid nomenclature". PeerJ. 5: e3782. doi:10.7717/peerj.3782. PMC 5602675. PMID 28929018.
- ↑ Madzia, D.; Cau, A. (2020). "Estimating the evolutionary rates in mosasauroids and plesiosaurs: discussion of niche occupation in Late Cretaceous seas". PeerJ. 8: e8941. doi:10.7717/peerj.8941. PMC 7164395. PMID 32322442.
- 1 2 Harrell Jr., T.L.; Pérez-Huerta, A.; Suarez, C.A. (2016). "Endothermic mosasaurs? Possible thermoregulation of Late Cretaceous mosasaurs (Reptilia, Squamata) indicated by stable oxygen isotopes in fossil bioapatite in comparison with coeval marine fish and pelagic seabirds". Palaeontology. 59 (3): 351–363. Bibcode:2016Palgy..59..351H. doi:10.1111/pala.12240. S2CID 130190966.
- ↑ Tattersall, G.J.; Leite, C.A.C.; Sanders, C.E.; Cadena, V.; Andrade, D.V.; Abe, A.S.; Milsom, W.K. (2016). "Seasonal reproductive endothermy in tegu lizards". Science Advances. 2 (1): e1500951. Bibcode:2016SciA....2E0951T. doi:10.1126/sciadv.1500951. PMC 4737272. PMID 26844295.
- ↑ Greene, C.C. (2018). Osteohistology And Skeletochronology Of An Ontogenetic Series Of Clidastes (Squamata: Mosasauridae): Growth And Metabolism In Basal Mosasaurids (MS). Fort Hays State University.
- ↑ Carpenter, J.A. (2017). Locomotion and skeletal morphology of Late Cretaceous mosasaur, Tylosaurus proriger (BS). Georgia Southern University.
- ↑ Massare, J (1988). "Swimming capabilities of Mesozoic marine reptiles: Implications for method of predation". Paleobiology. 14 (2): 187–205. Bibcode:1988Pbio...14..187M. doi:10.1017/S009483730001191X. S2CID 85810360.
- 1 2 3 4 5 6 Konishi, T.; Newbrey, M.; Caldwell, M. (2014). "A small, exquisitely preserved specimen of Mosasaurus missouriensis (Squamata, Mosasauridae) from the upper Campanian of the Bearpaw Formation, western Canada, and the first stomach contents for the genus". Journal of Vertebrate Paleontology. 34 (4): 802–819. Bibcode:2014JVPal..34..802K. doi:10.1080/02724634.2014.838573. S2CID 86325001.
- 1 2 Larson, N.L. (2010), Enchoteuthididae, giant squids from the Upper Cretaceous of the Western Interior In: 16th Annual Tate Conference, June 4–6, 2010: pp. 66-79.
- 1 2 Kauffman, E.G. (2004). "Mosasaur Predation on Upper Cretaceous Nautiloids and Ammonites from the United States Pacific Coast" (PDF). PALAIOS. 19 (1): 96–100. Bibcode:2004Palai..19...96K. doi:10.1669/0883-1351(2004)019<0096:MPOUCN>2.0.CO;2. S2CID 130690035.
- 1 2 3 Cicimurri, D.J.; Everhart, M.D. (2001). "An Elasmosaur with Stomach Contents and Gastroliths from the Pierre Shale (Late Cretaceous) of Kansas". Transactions of the Kansas Academy of Science. 104 (3 & 4): 129–143. doi:10.1660/0022-8443(2001)104[0129:aewsca]2.0.co;2. S2CID 86037286.
- ↑ Everhart, M.J. (2008). "Museum of Geology, South Dakota School of Mines". Oceans of Kansas. Archived from the original on September 27, 2020.
- ↑ Martin, J.E.; Bjork, P.R. (1987). Martin, J.E.; Ostrander, G.E. (eds.). "Gastric residues associated with a mosasaur from the Late Cretaceous (Campanian) Pierre Shale in South Dakota". Papers in Vertebrate Paleontology in Honor of Morton Green: Dakoterra. 3: 68–72.
- ↑ Royal Sask Museum [@royalsaskmuseum] (March 6, 2020). "A map showing the position of Omācīw, the Tylosaurus skeleton, as it was found in the quarry near Lake Diefenbaker in 1995. Its bones were arranged almost as they were in life" (Tweet) – via Twitter.
- ↑ Everhart 2017, p. 147-148.
- ↑ Pasch, A.D.; May, K.C. (2001). "Taphonomy and paleoenvironment of a hadrosaur (Dinosauria) from the Matanuska Formation (Turonian) in South-Central Alaska". In Tanke, D.H.; Carpenter, K.; Skrepnick, M. W. (eds.). Mesozoic Vertebrate Life. Indiana University Press. pp. 219–236.
- ↑ Grigoriev, D.V.; Grabovskiy, A.A. (2020). "Arctic mosasaurs (Squamata, Mosasauridae) from the Upper Cretaceous of Russia". Cretaceous Research. 114 (2020): 104499. Bibcode:2020CrRes.11404499G. doi:10.1016/j.cretres.2020.104499. S2CID 219431991.
- ↑ Rothschild, B.M.; Martin, L.D. (2005). "Mosasaur ascending: the phytogeny of bends". Netherlands Journal of Geosciences. 84 (Special Issue 3): 341–344. Bibcode:2005NJGeo..84..341R. doi:10.1017/S0016774600021120.
- ↑ Carlsen, A.W. (2017). "Frequency of decompression illness among recent and extinct mammals and "reptiles": a review". The Science of Nature. 104 (7–8): 56. Bibcode:2017SciNa.104...56C. doi:10.1007/s00114-017-1477-1. PMID 28656350. S2CID 23194069.
- ↑ Rothschild, B.; Everhart, M.J. (2015). "Co-Ossification of Vertebrae in Mosasaurs (Squamata, Mosasauridae); Evidence of Habitat Interactions and Susceptibility to Bone Disease". Transactions of the Kansas Academy of Science. 118 (3&4): 265–275. doi:10.1660/062.118.0309. S2CID 83690496.
- ↑ Everhart, M.J. (2001). "Mosasaur Pathology". Oceans of Kansas. Archived from the original on December 5, 2023.
Cited books
- Ellis, R. (2003). Sea Dragons: Predators of the Prehistoric Oceans. University Press of Kansas. ISBN 978-0700613946.
- Everhart, M.J. (2017). Oceans of Kansas, Second Edition: A Natural History of the Western Interior Sea (Life of the Past). Indiana University Press. ISBN 978-0253026323.
- Leidy, J. (1873). Contributions to the extinct vertebrate fauna of the western interior territories: Report of the United States Geological Survey of the Territories. U.S. Government Printing Office. doi:10.5962/bhl.title.61805.
- Mann, J.; Conner, R.C.; Tyack, P.L.; Whitehead, H. (2000). Cetacean Societies: Field Studies of Dolphins and Whales. University of Chicago Press. ISBN 978-0226503417.
- Russell, D.A. (1967). Systematics and Morphology of American Mosasaurs (PDF). Vol. 23. Bulletin of the Peabody Museum of Natural History. ISBN 978-1933789446. Archived from the original (PDF) on June 24, 2016. Retrieved December 24, 2020.
