Heterobimetallic catalysis is an approach to catalysis that employs two different metals to promote a chemical reaction. Included in this definition are cases (Scheme 1) where: 1) each metal activates a different substrate (synergistic catalysis, used interchangeably with the terms "cooperative" and "dual" catalysis.[1]), 2) both metals interact with the same substrate, and 3) only one metal directly interacts with the substrate(s), while the second metal interacts with the first.[2]

In synergistic catalysis
Complexes of palladium catalyze cross-coupling of electrophiles with organometallic nucleophiles, including those derived from lithium, tin, zinc, and boron.[3] One example is Sonogashira coupling, where catalytic amount of copper salt (e.g. CuI) reacts with a terminal alkyne (the pronucleophile) under basic conditions to generate a copper acetylide, which transmetalates onto an arylpalladiumII halide, regenerating the copper halide. Reductive elimination from the arylpalladium acetylide yields the cross-coupled product.[2]
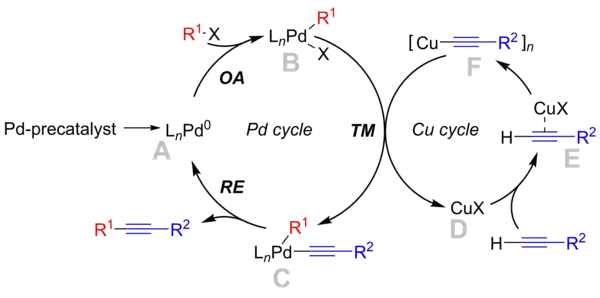
Other organic pronucleophiles are cross-coupled with arylpalladium halides in the following examples (Scheme 2):
1. Gold-catalyzed cyclization of allenoates followed by cross-coupling with aryl iodides yields 4-arylbutenolides[4]
2. Borylcupration of styrenes followed by palladium-catalyzed cross-coupling with aryl halides generates α-aryl-β-boromethyl functionalized arenes.[5][6] This reaction has been rendered diastereoselective in the case of cyclic styrenes,[7] and an enantioselective variant has also been developed.[8] Enantioselective hydroarylation of styrenes is accomplished similarly via a chiral copper hydride[9]
3. Asymmetric conjugate reduction-allylation of α,β-unsaturated ketones is achieved by Cu-H mediated reduction and subsequent allylation via a chiral PHOX-ligated palladium catalyst[10]
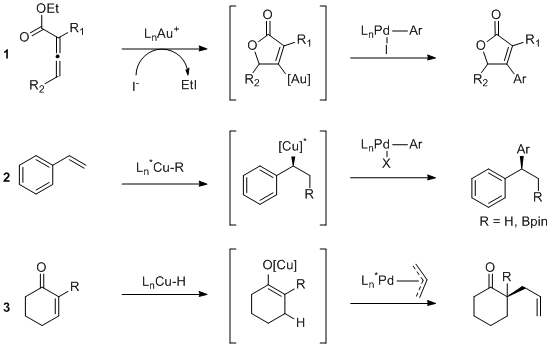
Also of note is the enantioselective allylation of activated nitriles (Scheme 3).[11] A chiral bisphosphine-ligated rhodium catalyst activates the alpha-keto-nitrile component as its corresponding enolate, which is intercepted by a π-allylpalladium complex to yield the α-allylated nitrile in high enantiomeric excess. In the absence of the rhodium catalyst no enantioselectivity is observed, whereas the reaction does not proceed in the absence of palladium.
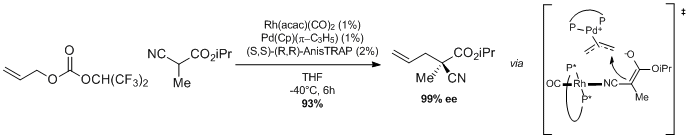
With preformed heterobimetallic catalysts
Catalyst systems in which both metal centers are contained in the same complex are also known (e.g. Shibasaki catalysts); further examples are provided below.
Ion-paired combinations of early and late transition metal complexes can simultaneously interact with a substrate as both Lewis acid and Lewis base.[2] For example, carbonylative ring expansion of epoxides (Scheme 4)[12][13][14] is accomplished by Lewis acid activation by cationic complexes of CrIII, TiIII or AlIII with simultaneous ring opening by the [Co(CO)4]− counterion. Carbonylation of the resultant alkylcobalt followed by lactonization releases the product.

A heterobimetallic bond-breaking process is also employed in the IPrCuFp-catalyzed C-H borylation system developed by Mankad (Scheme 5).[15] Bimetallic cleavage of the B-H bond in pinacolborane generates a copper hydride (IPrCu-H) and an iron boryl [(pin)B-Fp], the latter of which borylates unactivated arenes upon UV irradiation. Bimetallic reductive elimination of H2 from the combination of H-Fp and IPrCu-H restarts the catalytic cycle. The incorporation of copper into the catalyst is essential; C-H borylation using (pin)B-Fp alone is stoichiometric in iron due to dimerization of the HFp byproduct.
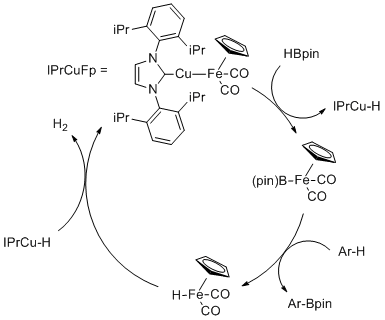
Heterobimetallic catalysts containing persistent M1-M2 bonds exhibit altered reactivity due to interaction of the two different metal centers. For example, allylic amination catalyzed by the binuclear complex [Cl2Ti(NtBuPPh2)2-/Pd(η3-CH2C(CH3)CH2)]+ is exceptionally rapid.[16] DFT studies suggest that a Pd→Ti dative interaction accelerates the typically slow reductive elimination step by withdrawing electron density from Pd in the transition state[17] (Scheme 6).
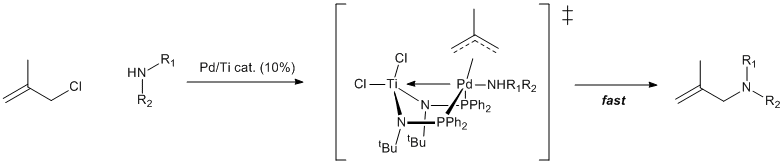
Silica-supported heterobimetallic tantalum iridium catalysts were shown exhibit drastically increased catalytic performances in H/D catalytic exchange reactions with respect to (i) monometallic analogues as well as (ii) homogeneous systems.[18] The key transition state in the C-H activation pathway, computed by DFT, involves (i) donation from the C-H σ orbital to an empty d orbital on the electrophilic early metal (Ta) together with (ii) backdonation from a filled d orbital arising from the late metal (Ir) to the C-H σ* orbital for nucleophilic assistance (Scheme 7). The calculations have shown that steric effects imparted by the ancillary ligands could result in enormous differences in C-H activation energy barriers (ca. 20 kcal/mol-1) in this heterobimetallic cooperative mechanism, indicating that metals accessibility has a drastic impact on the catalytic performances.[19]
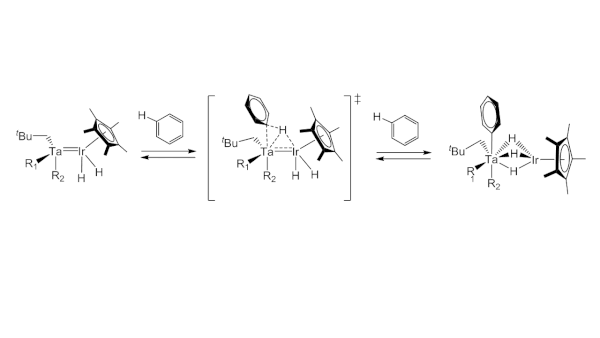
In photoredox catalysis
The combination of photoredox catalysis with traditional transition metal catalysis enables the use of visible light to drive challenging steps in a catalytic cycle.[20] For example, nickel-catalyzed aryl amination suffers from a difficult C-N reductive elimination step.[20] Hence instead of nickel, expensive palladium-based precatalysts are often used in combination with sterically encumbered phosphine ligands to facilitate reductive elimination.[20] A more recent approach employs an iridium-based photoredox catalyst to effect single-electron oxidation of the intermediate NiII-amido complex. The resulting NiIII-amido rapidly undergoes reductive elimination,[20] allowing the Ni-catalyzed aryl amination to proceed at room temperature without the use of phosphine ligands.
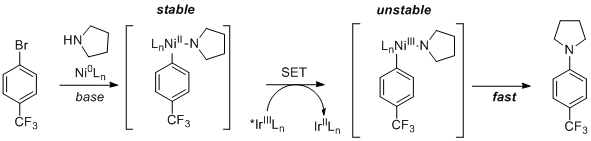
Biological significance
Enzymes containing two or more different metal centers are found in several important biological systems; for example, the Mo-Fe protein of nitrogenase[21] catalyzes the conversion of N2 to NH3 in nitrogen fixation. Of more relevance to human biology, Cu-Zn superoxide dismutase protects cells from oxidative stress by converting superoxide, O2−, to O2 and hydrogen peroxide[22]
References
- ↑ Schindler, C.; Jacobsen, E. (May 2013). "A New Twist on Cooperative Catalysis". Science. 340 (6136): 1052–1053. Bibcode:2013Sci...340.1052S. doi:10.1126/science.1238769. PMID 23723222. S2CID 35756570.
- 1 2 3 Pye, D.; Mankad, N. (March 2017). "Bimetallic catalysis for C–C and C–X coupling reactions". Chemical Science. 8 (3): 1705–1718. doi:10.1039/c6sc05556g. PMC 5933431. PMID 29780450.
- ↑ Organic Synthesis using Transition Metals Rod Bates ISBN 978-1-84127-107-1
- ↑ García-Domínguez, P.; Nevado, C. (March 2016). "Au–Pd Bimetallic Catalysis: The Importance of Anionic Ligands in Catalyst Speciation". J. Am. Chem. Soc. 138 (10): 3266–3269. doi:10.1021/jacs.5b10277. PMID 26952216.
- ↑ Semba, K.; Nakao, Y. (May 2014). "Arylboration of Alkenes by Cooperative Palladium/Copper Catalysis". J. Am. Chem. Soc. 136 (21): 7567–7570. doi:10.1021/ja5029556. PMID 24810227.
- ↑ Smith, K. B.; Logan, K. M.; You, W.; Brown, M. K. (August 2014). "Alkene carboboration enabled by synergistic catalysis". Chem. Eur. J. 20 (38): 12032–12036. doi:10.1002/chem.201404310. PMID 25113669.
- ↑ Logan, K. M.; Smith, K. B.; You, W.; Brown, M. K. (April 2015). "Copper/Palladium Synergistic Catalysis for the syn‐ and anti‐Selective Carboboration of Alkenes". Angew. Chem. Int. Ed. 54 (17): 5228–5231. doi:10.1002/anie.201500396. PMID 25727074.
- ↑ Jia, T.; Cao, P.; Wang, B.; Lou, Yazhou; Yin, X.; Wang, M.; Liao, J. (October 2015). "A Cu/Pd Cooperative Catalysis for Enantioselective Allylboration of Alkenes". J. Am. Chem. Soc. 137 (43): 13760–13763. doi:10.1021/jacs.5b09146. PMID 26458555.
- ↑ Friis, S. D.; Pirnot, M. T.; Buchwald, S. L. (June 2016). "Asymmetric Hydroarylation of Vinylarenes Using a Synergistic Combination of CuH and Pd Catalysis". J. Am. Chem. Soc. 138 (27): 8372–8375. doi:10.1021/jacs.6b04566. PMC 5010014. PMID 27346525.
- ↑ Nahra, F.; Mac'e, Y.; Riant, O. (February 2013). "Copper/Palladium‐Catalyzed 1,4 Reduction and Asymmetric Allylic Alkylation of α,β‐Unsaturated Ketones: Enantioselective Dual Catalysis". Angew. Chem. Int. Ed. 52 (11): 3208–3212. doi:10.1002/anie.201208612. PMID 23382027.
- ↑ Sawamura, M.; Sudoh, M.; Ito, Y. (April 1996). "An Enantioselective Two-Component Catalyst System: Rh−Pd-Catalyzed Allylic Alkylation of Activated Nitriles". J. Am. Chem. Soc. 118 (137): 3309–3310. doi:10.1021/ja954223e.
- ↑ Schmidt, J. A. R.; Lobkovsky, E. B.; Coates, G. W. (July 2005). "Chromium (III) octaethylporphyrinato tetracarbonylcobaltate: a highly active, selective, and versatile catalyst for epoxide carbonylation". J. Am. Chem. Soc. 127 (32): 11426–11435. doi:10.1021/ja051874u. PMID 16089471.
- ↑ Yutan, D. Y. L. Getzler; Mahadevan, V.; Lobkovsky, E. B.; Coates, G. W. (January 2002). "Synthesis of β-Lactones: A Highly Active and Selective Catalyst for Epoxide Carbonylation". J. Am. Chem. Soc. 124 (7): 1174–1175. doi:10.1021/ja017434u. PMID 11841278.
- ↑ Mulzer, M.; Whiting, B.; Coates, G. W. (June 2013). "Regioselective Carbonylation of trans-Disubstituted Epoxides to β-Lactones: A Viable Entry into syn-Aldol-Type Products". J. Am. Chem. Soc. 135 (30): 10930–10933. doi:10.1021/ja405151n. PMID 23790074.
- ↑ Mankad, N. (December 2013). "Non-Precious Metal Catalysts for C-H Borylation Enabled by Metal–Metal Cooperativity". Synlett. 25 (9): 1197–1201. doi:10.1055/s-0033-1340823. S2CID 196774326.
- ↑ Tsutsumi, H; Sunada, Y.; Shiota, Y.; Yoshizawa, K.; Nagashima, H. (March 2009). "Nickel(II), Palladium(II), and Platinum(II) η3-Allyl Complexes Bearing a Bidentate Titanium(IV) Phosphinoamide Ligand: A Ti←M2 Dative Bond Enhances the Electrophilicity of the π-Allyl Moiety". Organometallics. 28 (7): 1988–1991. doi:10.1021/om8011085.
- ↑ Walker, W. K.; Kay, B. M.; Michaelis, S.A.; Anderson, D. L.; Smith, S.J.; Ess, D. H.; Michaelis, D.J. (2015). "Origin of Fast Catalysis in Allylic Amination Reactions Catalyzed by Pd-Ti Heterobimetallic Complexes". Journal of the American Chemical Society. 137 (23): 7371–7378. doi:10.1021/jacs.5b02428. PMID 25946518.
- ↑ Lassalle, S.; Jabbour, R.; Schiltz, P.; Berruyer, P.; Todorova, T. K.; Veyre, L.; Gajan, D.; Lesage, A.; Thieuleux, C.; Camp, C. (2019). "Metal–Metal Synergy in Well-Defined Surface Tantalum–Iridium Heterobimetallic Catalysts for H/D Exchange Reactions". Journal of the American Chemical Society. 141 (49): 19321–19335. doi:10.1021/jacs.9b08311. PMID 31710215. S2CID 207944756.
- ↑ Del Rosal, I.; Lassalle, S.; Dinoi, C.; Thieuleux, C.; Maron, L.; Camp, C. (2021). "Mechanistic investigations via DFT support the cooperative heterobimetallic C–H and O–H bond activation across TaIr multiple bonds" (PDF). Dalton Transactions. 50: 504–510. doi:10.1039/D0DT03818K. PMID 33210676. S2CID 227064747.
- 1 2 3 4 Corcoran, E. B.; Pirnot, M. T.; Lin, S.; Dreher, S. D.; DiRocco, D. A.; Davies, I. W.; Buchwald, S. L. (July 2016). "Aryl amination using ligand-free Ni(II) salts and photoredox catalysis". Journal of the American Chemical Society. 353 (6296): 279–283. Bibcode:2016Sci...353..279C. doi:10.1126/science.aag0209. PMC 5027643. PMID 27338703.
- ↑ Burges BK, Lowe DJ (1996). "Mechanism of Molybdenum Nitrogenase". Chemical Reviews. 96 (7): 2983–3011. doi:10.1021/cr950055x. PMID 11848849.
- ↑ Richardson J, Thomas KA, Rubin BH, Richardson DC (Apr 1975). "Crystal structure of bovine Cu,Zn superoxide dismutase at 3 A resolution: chain tracing and metal ligands". Proceedings of the National Academy of Sciences of the United States of America. 72 (4): 1349–53. doi:10.1073/pnas.72.4.1349. PMC 432531. PMID 1055410..