Part of a series of articles on |
Molecular nanotechnology |
---|
Part of a series of articles on |
Nanotechnology |
---|
Impact and applications |
Nanomaterials |
Molecular self-assembly |
Nanoelectronics |
Nanometrology |
Molecular nanotechnology |
Mechanosynthesis is a term for hypothetical chemical syntheses in which reaction outcomes are determined by the use of mechanical constraints to direct reactive molecules to specific molecular sites. There are presently no non-biological chemical syntheses which achieve this aim. Some atomic placement has been achieved with scanning tunnelling microscopes.
Introduction
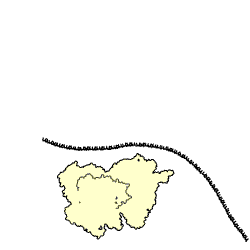
In conventional chemical synthesis or chemosynthesis, reactive molecules encounter one another through random thermal motion in a liquid or vapor. In a hypothesized process of mechanosynthesis, reactive molecules would be attached to molecular mechanical systems, and their encounters would result from mechanical motions bringing them together in planned sequences, positions, and orientations. It is envisioned that mechanosynthesis would avoid unwanted reactions by keeping potential reactants apart, and would strongly favor desired reactions by holding reactants together in optimal orientations for many molecular vibration cycles. In biology, the ribosome provides an example of a programmable mechanosynthetic device.
A non-biological form of mechanochemistry has been performed at cryogenic temperatures using scanning tunneling microscopes.[1] So far, such devices provide the closest approach to fabrication tools for molecular engineering. Broader exploitation of mechanosynthesis awaits more advanced technology for constructing molecular machine systems, with ribosome-like systems as an attractive early objective.
Much of the excitement regarding advanced mechanosynthesis regards its potential use in assembly of molecular-scale devices. Such techniques appear to have many applications in medicine, aviation, resource extraction, manufacturing and warfare.
Most theoretical explorations of advanced machines of this kind have focused on using carbon, because of the many strong bonds it can form, the many types of chemistry these bonds permit, and utility of these bonds in medical and mechanical applications. Carbon forms diamond, for example, which if cheaply available, would be an excellent material for many machines.
It has been suggested, notably by K. Eric Drexler, that mechanosynthesis will be fundamental to molecular manufacturing based on nanofactories capable of building macroscopic objects with atomic precision. The potential for these has been disputed, notably by Nobel Laureate Richard Smalley (who proposed and then critiqued an unworkable approach based on "Smalley fingers").[2]
The Nanofactory Collaboration,[3] founded by Robert Freitas and Ralph Merkle in 2000, is a focused ongoing effort involving 23 researchers from 10 organizations and 4 countries that is developing a practical research agenda[4] specifically aimed at positionally controlled diamond mechanosynthesis and diamondoid nanofactory development.
In practice, getting exactly one molecule to a known place on the microscope's tip is possible, but has proven difficult to automate. Since practical products require at least several hundred million atoms, this technique has not yet proven practical in forming a real product.
The goal of one line of mechanoassembly research focuses on overcoming these problems by calibration, and selection of appropriate synthesis reactions. Some suggest attempting to develop a specialized, very small (roughly 1,000 nanometers on a side) machine tool that can build copies of itself using mechanochemical means, under the control of an external computer. In the literature, such a tool is called an assembler or molecular assembler. Once assemblers exist, geometric growth (directing copies to make copies) could reduce the cost of assemblers rapidly. Control by an external computer should then permit large groups of assemblers to construct large, useful projects to atomic precision. One such project would combine molecular-level conveyor belts with permanently mounted assemblers to produce a factory.
In part to resolve this and related questions about the dangers of industrial accidents and popular fears of runaway events equivalent to Chernobyl and Bhopal disasters, and the more remote issue of ecophagy, grey goo and green goo (various potential disasters arising from runaway replicators, which could be built using mechanosynthesis) the UK Royal Society and UK Royal Academy of Engineering in 2003 commissioned a study to deal with these issues and larger social and ecological implications, led by mechanical engineering professor Ann Dowling. This was anticipated by some to take a strong position on these problems and potentials —– and suggest any development path to a general theory of so-called mechanosynthesis. However, the Royal Society's nanotech report did not address molecular manufacturing at all, except to dismiss it along with grey goo.
Current technical proposals for nanofactories do not include self-replicating nanorobots, and recent ethical guidelines would prohibit development of unconstrained self-replication capabilities in nanomachines.[5][6]
Diamond mechanosynthesis
There is a growing body of peer-reviewed theoretical work on synthesizing diamond by mechanically removing/adding hydrogen atoms [7] and depositing carbon atoms [8][9][10][11][12][13] (a process known as diamond mechanosynthesis or DMS[14]). For example, the 2006 paper in this continuing research effort by Freitas, Merkle and their collaborators reports that the most-studied mechanosynthesis tooltip motif (DCB6Ge) successfully places a C2 carbon dimer on a C(110) diamond surface at both 300 K (room temperature) and 80 K (liquid nitrogen temperature), and that the silicon variant (DCB6Si) also works at 80 K but not at 300 K. These tooltips are intended to be used only in carefully controlled environments (e.g., vacuum). Maximum acceptable limits for tooltip translational and rotational misplacement errors are reported in paper III—tooltips must be positioned with great accuracy to avoid bonding the dimer incorrectly. Over 100,000 CPU hours were invested in this study.
The DCB6Ge tooltip motif, initially described at a Foresight Conference in 2002, was the first complete tooltip ever proposed for diamond mechanosynthesis and remains the only tooltip motif that has been successfully simulated for its intended function on a full 200-atom diamond surface. Although an early paper gives a predicted placement speed of 1 dimer per second for this tooltip, this limit was imposed by the slow speed of recharging the tool using an inefficient recharging method[10] and is not based on any inherent limitation in the speed of use of a charged tooltip. Additionally, no sensing means was proposed for discriminating among the three possible outcomes of an attempted dimer placement—deposition at the correct location, deposition at the wrong location, and failure to place the dimer at all—because the initial proposal was to position the tooltip by dead reckoning, with the proper reaction assured by designing appropriate chemical energetics and relative bond strengths for the tooltip-surface interaction.
More recent theoretical work[15] analyzes a complete set of nine molecular tools made from hydrogen, carbon and germanium able to (a) synthesize all tools in the set (b) recharge all tools in the set from appropriate feedstock molecules and (c) synthesize a wide range of stiff hydrocarbons (diamond, graphite, fullerenes, and the like). All required reactions are analyzed using standard ab initio quantum chemistry methods.
Further research [16] to consider alternate tips will require time-consuming computational chemistry and difficult laboratory work. In the early 2000s, a typical experimental arrangement was to attach a molecule to the tip of an atomic force microscope, and then use the microscope's precise positioning abilities to push the molecule on the tip into another on a substrate. Since the angles and distances can be precisely controlled, and the reaction occurs in a vacuum, novel chemical compounds and arrangements are possible.
History
The technique of moving single atoms mechanically was proposed by Eric Drexler in his 1986 book The Engines of Creation.
In 1989, researchers at IBM's Zürich Research Institute successfully spelled the letters "IBM" in xenon atoms on a cryogenic copper surface, grossly validating the approach.[1][17] Since then, a number of research projects have undertaken to use similar techniques to store computer data in a compact fashion. More recently the technique has been used to explore novel physical chemistries, sometimes using lasers to excite the tips to particular energy states, or examine the quantum chemistry of particular chemical bonds.
In 1999, an experimentally proved methodology called feature-oriented scanning[18][19] (FOS) was suggested. The feature-oriented scanning methodology allows precisely controlling the position of the probe of a scanning probe microscope (SPM) on an atomic surface at room temperature. The suggested methodology supports fully automatic control of single- and multiprobe instruments in solving tasks of mechanosynthesis and bottom-up nanofabrication.
In 2003, Oyabu et al.[20] reported the first instance of purely mechanical-based covalent bond-making and bond-breaking, i.e., the first experimental demonstration of true mechanosynthesis—albeit with silicon rather than carbon atoms.
In 2005, the first patent application on diamond mechanosynthesis [21] was filed.
In 2008, a $3.1 million grant was proposed[22] to fund the development of a proof-of-principle mechanosynthesis system.
In 2013, IBM made A Boy and His Atom, a short animated film using atoms.[23]
See also molecular nanotechnology, a more general explanation of the possible products, and discussion of other assembly techniques.
References
- 1 2 Eigler, D. M.; Schweizer, E. K. (April 1990). "Positioning single atoms with a scanning tunnelling microscope". Nature. 344 (6266): 524–526. doi:10.1038/344524a0. ISSN 0028-0836.
- ↑ Baum, Rudy (2003-12-01). "Drexler and Smalley make the case for and against 'molecular assemblers'". Chemical & Engineering News. 81 (48): 27–42. ISSN 0009-2347.
- ↑ Nanofactory Collaboration. Molecularassembler.com. Retrieved on 2011-07-23.
- ↑ Nanofactory Technical Challenges. Molecularassembler.com. Retrieved on 2011-07-23.
- ↑ Molecular Nanotechnology Guidelines. Foresight.org. Retrieved on 2011-07-23.
- ↑ N04FR06-p.15.pmd. (PDF) . Retrieved on 2011-07-23.
- ↑ Temelso, Berhane; Sherrill, C. David; Merkle, Ralph C.; Freitas, Robert A. (2006). "High-level Ab Initio Studies of Hydrogen Abstraction from Prototype Hydrocarbon Systems" (PDF). The Journal of Physical Chemistry A. 110 (38): 11160–11173. Bibcode:2006JPCA..11011160T. CiteSeerX 10.1.1.154.7331. doi:10.1021/jp061821e. PMID 16986851.
- ↑ Merkle, RC; Freitas Jr, RA (2003). "Theoretical Analysis of a Carbon-Carbon Dimer Placement Tool for Diamond Mechanosynthesis" (PDF). Journal of Nanoscience and Nanotechnology. 3 (4): 319–24. doi:10.1166/jnn.2003.203. PMID 14598446.
- ↑ Peng, Jingping; Freitas, Robert A.; Merkle, Ralph C. (2004). "Theoretical Analysis of Diamond Mechanosynthesis. Part I. Stability of C2 Mediated Growth of Nanocrystalline Diamond C(110) Surface" (PDF). Journal of Computational and Theoretical Nanoscience. 1: 62–70. doi:10.1166/jctn.2004.007. Archived from the original (PDF) on 2009-03-16.
- 1 2 Mann, David J.; Peng, Jingping; Freitas, Robert A.; Merkle, Ralph C. (2004). "Theoretical Analysis of Diamond Mechanosynthesis. Part II. C2 Mediated Growth of Diamond C(110) Surface via Si/Ge-Triadamantane Dimer Placement Tools" (PDF). Journal of Computational and Theoretical Nanoscience. 1: 71–80. doi:10.1166/jctn.2004.008.
- ↑ Sourina, Olga; Korolev, Nikolay (2005). "Design and Analysis of a Molecular Tool for Carbon Transfer in Mechanosynthesis" (PDF). Journal of Computational and Theoretical Nanoscience. 2 (4): 492–498. Bibcode:2005JCTN....2..492S. doi:10.1166/jctn.2005.003.
- ↑ De Federico, Miguel; Jaime, Carlos (2006). "Theoretical Analysis of Diamond Mechanosynthesis. Part III. Positional C2 Deposition on Diamond C(110) Surface using Si/Ge/Sn-based Dimer Placement Tools" (PDF). Journal of Computational and Theoretical Nanoscience. 3 (6): 874–879. arXiv:cond-mat/0605239. Bibcode:2006JCTN....3..624S. doi:10.1166/jctn.2006.003.
- ↑ Yin, Zhi-Xiang; Cui, Jian-Zhong; Liu, Wenbin; Shi, Xiao-Hong; Xu, Jin (2007). "Horizontal Ge-Substituted Polymantane-Based C2 Dimer Placement Tooltip Motifs for Diamond Mechanosynthesis" (PDF). Journal of Computational and Theoretical Nanoscience. 4 (7): 1243–1248. doi:10.1166/jctn.2007.004.
- ↑ Diamond Mechanosynthesis. Molecularassembler.com. Retrieved on 2011-07-23.
- ↑ Freitas Jr., Robert A.; Merkle, Ralph C. (2008). "A Minimal Toolset for Positional Diamond Mechanosynthesis" (PDF). Journal of Computational and Theoretical Nanoscience. 5 (7): 760–861. Bibcode:2008JCTN....5..760F. doi:10.1166/jctn.2008.2531.
- ↑ Speeding the development of molecular nanotechnology. www.foresight.org
- ↑ Shankland, S. (28 September 2009). "IBM's 35 atoms and the rise of nanotech". CNET. Retrieved 2023-05-09.
- ↑ R. V. Lapshin (2004). "Feature-oriented scanning methodology for probe microscopy and nanotechnology" (PDF). Nanotechnology. 15 (9): 1135–1151. Bibcode:2004Nanot..15.1135L. doi:10.1088/0957-4484/15/9/006. ISSN 0957-4484. (Russian translation is available).
- ↑ R. V. Lapshin (2011). "Feature-oriented scanning probe microscopy". In H. S. Nalwa (ed.). Encyclopedia of Nanoscience and Nanotechnology (PDF). Vol. 14. USA: American Scientific Publishers. pp. 105–115. ISBN 978-1-58883-163-7.
- ↑ Oyabu, Noriaki; Custance, ÓScar; Yi, Insook; Sugawara, Yasuhiro; Morita, Seizo (2003). "Mechanical Vertical Manipulation of Selected Single Atoms by Soft Nanoindentation Using Near Contact Atomic Force Microscopy". Physical Review Letters. 90 (17): 176102. Bibcode:2003PhRvL..90q6102O. doi:10.1103/PhysRevLett.90.176102. PMID 12786084.
- ↑ Robert A. Freitas Jr., "A Simple Tool for Positional Diamond Mechanosynthesis, and its Method of Manufacture," U.S. Patent 7,687,146, issued 30 March 2010 HTML copy Retrieved on 2011-07-23.
- ↑ Digital Matter?: Towards Mechanised Mechanosynthesis Archived 2011-11-04 at the Wayback Machine. Gow.epsrc.ac.uk. Retrieved on 2011-07-23.
- ↑ "A Boy And His Atom". IBM Research. May 1, 2013. Retrieved December 29, 2015.