In behavioral biology, the optomotor response is an innate, orienting behavior evoked by whole-field visual motion and is common to fish and insects during locomotion, such as swimming, walking and flying.[1][2][3][4][5][6][7][8][9] The optomotor response has algorithmic properties such that the direction of the whole-field coherent motion dictates the direction of the behavioral output (e.g., leftward visual stimuli lead to turning left, and rightward visual stimuli lead to turning right). For instance, when zebrafish larvae are presented with a sinusoidal black and white grating pattern, the larvae will turn and swim in the direction of the perceived motion.[10]
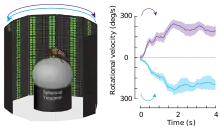
Purpose
The optomotor response is essential for animals to correct unplanned course perturbations while navigating through their environment, such as current shifts around a swimming fish or air gusts around flying insects. The response is rapid and instinctual, with pure delay times of just 20-40ms for fruit flies in flight.[11]
The optomotor response is a central feature of a fly's flight control system: flies subject to unplanned apparent self-motion move to minimize the resultant optic flow (retinal movement patterns) and correct involuntary deviations from course.[1][12][13][14][15] In their natural environments, full-field optic flow patterns are elicited by distinct flight maneuvers; for instance, rotational optic flow is generated by body rotation during hovering, whereas expansion optic flow is elicited by body translation during straight flight. As such, flies respond to panoramic retinal patterns of visual expansion with robust steering maneuvers away from the expansion point (mimicking an approaching object) to avoid collisions and maintain upwind flight postures.[16]
Research Applications
The optomotor response is frequently used as a behavioral assay. In zebrafish, the optomotor response is frequently used as a metric of visual performance as it can be reliably evoked from 7 days post fertilization throughout adulthood.[4][7] The contrast and wavelength (color) of the stripes can be manipulated to assess the specific properties of their visual system, such as testing the contribution of color to motion detection.[17] In flies, the optomotor response is used to understand the functional properties of neural circuits in the context of a specific behavior and examine the sensorimotor transformations underlying that behavior. To describe the physiological or behavioral properties of the optomotor response, researchers typically vary the spatial period of projected visual patterns and their velocity. The stimulus regime is often composed of periods of open-loop large-field rotation or expansion stimuli alternating with periods of closed-loop stripe fixation in which the animal has control of the position of a single vertical bar.
Characteristics
Both behavioral and physiological optomotor responses have distinct tuning curves for the temporal, spatial and contrast structure of moving images.[5][18][19] The magnitude and time-course of the optomotor response to optic flow depends on the temporal frequency of image motion, the spatial period of the display pattern, the periodic contrast and the spatial organization of the stimulus, e.g. rotation or expansion. Typically, low spatial-period patterns (i.e. narrow stripes) produce weaker steering responses than high spatial-period patterns (i.e. wide stripes).[16] The strength of the optomotor response to different temporal frequencies for are state-dependent: stationary flies have a peak temporal frequency optima around 1 Hz,[16][20] while walking flies have a peak behavior response to optic flow between 1–4 Hz [5][21][20][16] and the optimal frequency during flight is much faster, between 3–12 Hz [22][20][16]
References
- 1 2 Götz, Karl Georg (June 1968). "Flight control in Drosophila by visual perception of motion". Kybernetik. 4 (6): 199–208. doi:10.1007/BF00272517. PMID 5731498. S2CID 24070951.
- ↑ Borst, A; Bahde, S (1993). "Comparison between the movement detection systems underlying the optomotor and the landing response in the housefly". Biol. Cybern. 56 (4): 217–224. doi:10.1007/BF00365216. S2CID 44797496.
- ↑ Reichardt, W (1966). "Detection of single quanta by the compound eye of the fly Mollusca". Proceedings of the International Symposium on the Functional Organization of the Compound Eye: 267–289.
- 1 2 Hu, M; Easter, SS (1999). "Retinal neurogenesis: the formation of the initial central patch of postmitotic cells". Dev Biol. 207 (2): 309–21. doi:10.1006/dbio.1998.9031. PMID 10068465.
- 1 2 3 Buchner, E (1984). "Behavioral analysis of spatial vision in insects". Photoreception and Vision in Invertebrates: 522–561.
- ↑ Wehrhahn, C (1985). Visual guidance of flies during flight. Oxford: Pergamon Press. pp. 673–684.
- 1 2 Schmitt, EA; Dowling, JE (1994). "Early eye morphogenesis in the zebrafish, Brachydanio rerio". J Comp Neurol. 344 (4): 532–42. doi:10.1002/cne.903440404. PMID 7929890. S2CID 29909206.
- ↑ Arnold, G. P. (1974). "Rheotropism in fishes". Biological Reviews. 49 (4): 515–576. doi:10.1111/j.1469-185X.1974.tb01173.x. PMID 4616732. S2CID 30755969.
- ↑ Lehrer, M. (1993). "Spatial vision in the honeybee: the use of different cues in different tasks". Vision Research. 34 (18): 2363–2385. doi:10.1016/0042-6989(94)90282-8. PMID 7975277. S2CID 40688546.
- ↑ Fleisch, VC; Neuhauss, SCF (2006). "Visual Behavior in Zebrafish". Zebrafish. 3 (2): 191–201. doi:10.1089/zeb.2006.3.191. PMID 18248260.
- ↑ Theobald, JC; Ringach, DL; Frye, MA (2010). "Dynamics of optomotor responses in drosophila to perturbations in optic flow". Journal of Experimental Biology. 213 (8): 1366–1375. doi:10.1242/jeb.037945. PMC 2846167. PMID 20348349.
- ↑ Reichardt, W; Poggio, T (1976). "Visual control of orientation behaviour in the fly: Part I. A quantitative analysis". Quarterly Reviews of Biophysics. 9 (3): 211–275. doi:10.1017/S0033583500002523. PMID 790441. S2CID 45258548.
- ↑ Collett, TS (1980). "Angular tracking and the optomotor response an analysis of visual reflex interaction in a hoverfly". Jour of Comp Physiol. 140 (2): 145–158. doi:10.1007/BF00606306. S2CID 44050294.
- ↑ Gotz, Karl G.; Wehrhahn, Christian (November 1984). "Optomotor control of the force of flight in Drosophila and Musca". Biological Cybernetics. 51 (2): 129–134. doi:10.1007/BF00357926. ISSN 1432-0770. S2CID 33981643.
- ↑ Lehmann, Fritz-Olaf; Mronz, Markus (1 July 2008). "The free-flight response of Drosophila to motion of the visual environment". Journal of Experimental Biology. 211 (13): 2026–2045. doi:10.1242/jeb.008268. ISSN 0022-0949. PMID 18552291.
- 1 2 3 4 5 Frye, Mark A.; Condro, Michael; Chow, Dawnis M.; Duistermars, Brian J. (15 September 2007). "The spatial, temporal and contrast properties of expansion and rotation flight optomotor responses in Drosophila". Journal of Experimental Biology. 210 (18): 3218–3227. doi:10.1242/jeb.007807. ISSN 0022-0949. PMID 17766299.
- ↑ Krauss, Andrea; Neumeyer, Christa (1 May 2003). "Wavelength dependence of the optomotor response in zebrafish (Danio rerio)". Vision Research. 43 (11): 1275–1284. doi:10.1016/S0042-6989(03)00090-7. ISSN 0042-6989. PMID 12726833.
- ↑ Götz, Karl Georg (1 September 1975). "The optomotor equilibrium of theDrosophila navigation system". Journal of Comparative Physiology. 99 (3): 187–210. doi:10.1007/BF00613835. ISSN 1432-1351. S2CID 23177851.
- ↑ Warrant, E. J.; Bidweii, N. J.; O'Carroll, D. C. (July 1996). "Insect motion detectors matched to visual ecology". Nature. 382 (6586): 63–66. Bibcode:1996Natur.382...63O. doi:10.1038/382063a0. ISSN 1476-4687. PMID 21638927. S2CID 4303068.
- 1 2 3 Chiappe, M. Eugenia; Seelig, Johannes D.; Reiser, Michael B.; Jayaraman, Vivek (24 August 2010). "Walking Modulates Speed Sensitivity in Drosophila Motion Vision". Current Biology. 20 (16): 1470–1475. doi:10.1016/j.cub.2010.06.072. ISSN 0960-9822. PMC 4435946. PMID 20655222.
- ↑ Götz, Karl Georg; Wenking, Hans (1 September 1973). "Visual control of locomotion in the walking fruitflyDrosophila". Journal of Comparative Physiology. 85 (3): 235–266. doi:10.1007/BF00694232. ISSN 1432-1351. S2CID 12552378.
- ↑ Dickinson, Michael H.; Straw, Andrew D.; Rohrseitz, Nicola; Fry, Steven N. (15 April 2009). "Visual control of flight speed in Drosophila melanogaster". Journal of Experimental Biology. 212 (8): 1120–1130. doi:10.1242/jeb.020768. ISSN 0022-0949. PMID 19329746.