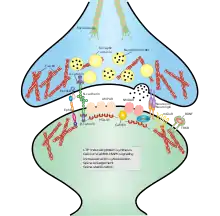
Synaptic stabilization is crucial in the developing and adult nervous systems and is considered a result of the late phase of long-term potentiation (LTP). The mechanism involves strengthening and maintaining active synapses through increased expression of cytoskeletal and extracellular matrix elements and postsynaptic scaffold proteins, while pruning less active ones. For example, cell adhesion molecules (CAMs) play a large role in synaptic maintenance and stabilization. Gerald Edelman discovered CAMs and studied their function during development, which showed CAMs are required for cell migration and the formation of the entire nervous system.[1][2] In the adult nervous system, CAMs play an integral role in synaptic plasticity relating to learning and memory.[3]
Types of CAMs
SynCAMs
Synaptic cell adhesion molecules (CAMs) play a crucial role in axon pathfinding and synaptic establishment between neurons during neurodevelopment and are integral members in many synaptic processes including the correct alignment of pre- and post-synaptic signal transduction pathways, vesicular recycling in regards to endocytosis and exocytosis, integration of postsynaptic receptors and anchoring to the cytoskeleton to ensure stability of synaptic components [4]
SynCAM’s (also known as Cadm or nectin-like molecules) are a specific type of synaptic CAM found in vertebrates that promotes growth and stabilization of excitatory (not inhibitory) synapses. SynCAM’s are localized primarily in the brain at both pre- and postsynaptic sites and their structures consist of intracellular FERM and PDZ binding domains, a single transmembrane domain, and three extracellular Ig-domains. During neurodevelopment, SynCAMs such as SynCAM1 act as “contact sensors” of axonal growth cones accumulating rapidly when axo-dendritic connections are made and helping to form a stable adhesion complex.[5]
synCAM1 along with neuroligin are the two CAM’s known to be sufficient to initiate the formation of presynaptic terminals, as addition of synCAM1 to media of co-cultured neuronal and non-neuronal cells lead to the establishment of presynaptic terminals. Homophillic binding of two synCAM1 molecules on the filopodia of axonal growth cone and dendritic spine allow for initial contact between pre- and postsynaptic cell to be made. [6]
synCAMs belong to the Ig superfamily of proteins. The cytosolic PDZ domains of synCAMs imbedded in the post-synaptic membrane interact with post-synaptic scaffold protein PSD-95 which helps anchor the complex to the underlying cytoskeleton. [7]
Cadherin-catenin
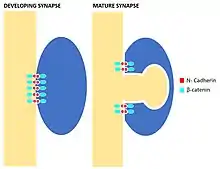
Cadherins are calcium- dependent, homophilic cell adhesion molecules that form complexes with cytosolic partners known as catenins.[8] Components of this complex bind to a number of different scaffolding proteins, phosphotases, kinases, and receptors.[9] Classical cadherins have five extracellular repeating structures which bind calcium, a single transmembrane domain, and an intracellular tail with a distal cytosolic domain that binds a catenin partner.[9][10] Recent work has implicated the cadherin-catenin complex in a number of different central nervous system processes such as synaptic stabilization and plasticity.[8][9][10]
Many cadherins in the central nervous system exhibit distinct spatial and temporal expression patterns.[9] For example, N-cadherin is widely expressed at the developing synapse and later remains near the mature active zone implicating that this complex may be well-suited to provide a link between structural changes and synaptic stability.[9] In fact, local synaptic activity changes impact the expression of the cadherin-catenin complexes.[9] An increase in activity at a particular spine leads to the dimerization of N-cadherin which is then cleaved leading the repression of CBP/CREB transcription.[9] This repression has many developmental and plasticity related implications.
In the case of dendritic spine formation and pruning, a competition hypothesis has been proposed and corroborated.[11][12] This hypothesis suggests that relative levels of cadherin-catenin complexes, which are distributed amongst spines in a local area in an activity-dependent manner, determines the fate of individual spines. That is, the inter-spine competition for β-catenin determines whether a spine will be matured (increased number of complexes) or pruned (decreased number of complexes).[12] This is a critical mechanism during the refinement of cortical circuitry that occurs throughout development.[11]
Nectin
Nectins are a distinct family of cell adhesion molecules. These CAMs are involved in the initial contact of presynaptic and postsynaptic neuronal processes during synapse formation. There are only four well characterized nectins at the synapse, they are Nectin-1, 2, 3, and 4.[13] All membrane-bound nectins possess an extracellular region with three immunoglobulin-like loops. The furthest loop from the membrane is called the V-type loop and the two loops more interior are C2-type loops. Multiple nectins on one cell membrane will bind together at the V-type loop to form a cluster of nectin proteins, a process called cis-clustering. When two cells possessing individual cis-clusters come into contact they form a strong complex called a trans-interaction which provides adhesion and, in some cases, signaling between the two cells.[14]
The most robust knowledge of nectin’s role in synaptic stabilization comes from the synapses made between mossy fiber terminals and pyramidal cell dendrites in the CA3 region of the hippocampus.[15] The nectins involved in formation and stabilization of this synapse are Nectin-1 and Nectin-3 which protrude from the plasma membrane of the postsynaptic cell and presynaptic cell, respectively, forming heterophilic extracellular contacts. The intracellular domain of all nectins directly bind to a protein called L-Afadin. L-Afadin is an actin binding protein that binds to the F-actin of the actin cytoskeleton. In this way, nectins form ridged connections of the cells actin architecture allowing for the synapse to develop in a controlled and stable environment.[16]
As synapses mature in the CA3 region, nectins and cadherins, which affiliate closely with one another in synaptic stabilization, are shifted to the periphery of the active zone and form the puncta adherens junction (PAJ). The PAJ functions much like the adherens junctions in epithelial tissues. The displacement of these CAMs and the formation of this junction provides the nascent synaptic membranes room to interact and mature while partitioning off the surrounding membrane and providing cytoskeletal fixation.[14]
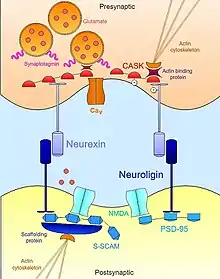
Neurexin-neuroligin
Neurexin-Neuroligin interactions help establish the trans-synaptic functional asymmetry essential for the stabilization and maintenance of proper synaptic transmission.[17] Presynaptic neurexin and its postsynaptic binding partner, neuroligin, complex early in neural development and are both known to be potent inducers of synaptogenesis.[18] Non-neuronal cells that artificially express neurexin are sufficient to mobilize post-synaptic specializations in co-cultured neurons;[19] neuroligin-expressing cells are likewise able to induce markers of pre-synaptic differentiation in neighboring neurons.[20][21] However, while both play an important role in synaptogenesis, these cell adhesion molecule are not necessary for formation of neuronal connections during development.[22] A triple knockout mouse mutant of either neurexins or neuroligins exhibit a normal number of synapses but express an embryonic lethal phenotype due to impairment of normal synaptic transmission.[23] Therefore, they are not necessary for synapse formation per se but are essential for the maturation and integration of synapses into the functional circuits necessary for survival.
Beyond their extracellular contact with each other, neurexins and neuroligins also bind intracellularly to a vast network of adaptor proteins and scaffolding structures, which in concert with the actin cytoskeleton, help localize necessary components of synaptic transmission. For example, the first neuroligin (NLGN1) discovered was identified by its PDZ domain which binds to PSD95, a well-known a scaffold protein at glutamatergic synapses that functionally links NMDA receptors to the proper post-synaptic locale.[21][24] Similarly, another isoform of neuroligin (NLGN2) interacts with gephyrin, a scaffolding protein specific to GABA-ergic synapses, and is responsible for activation of the synaptic adapator protein collybistin.[25] In the case of neurexins, their intracellular binding interactions are equally as important in recruiting the essential machinery for synaptic transmission at the active zone. Like neuroligins, neurexins possess a PDZ-domain that associates with CASK (Calcium-calmodulin-dependent protein kinase).[24] In addition to phosphorylating itself and neurexin, CASK promotes interactions between neurexins and actin binding proteins, thus providing a direct link by which neurexin can modulate cytoskeletal dynamics that is essential for synaptic stability and plasticity. Neurexin can also bind synaptotagmin, a protein embedded in the membrane of synaptic vesicles, and can also promote associations with voltage-gated calcium channel which mediate the ion flux required for neurotransmitter exocytosis upon synaptic stimulation.[26][23] In this way, neurexin and neuroligin coordinate the morphological and functional aspects of the synapse which in turn permits nascent, immature contacts to stabilize into full-fledged functional platforms for neurotransmission.
Ephrin-Eph signaling
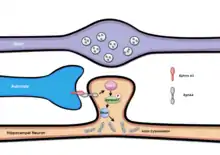
Non-traditional adhesion molecules, such as the ephrins, also help stabilize synaptic contacts. Eph receptors and their membrane bound ligands, the ephrins, are involved in a variety of cellular processes during development and maturation including axon guidance, neuronal migration, synaptogenesis, and axon pruning.[27][28] In the hippocampus, dendritic spine morphology may be regulated by astrocytes via bi-directional ephrin/EphA signaling.[29] Astrocytes and their processes express ephrin A3, whereas the EphA4 receptor is enriched in hippocampal neurons. This interaction, mediated by ephrin A3/EphA4 signaling, induces the recruitment and activation of cyclin-dependent kinase 5 (Cdk5), which then phosphorylates the guanine exchange factor (GEF), ephexin1.[30] Phosphorylated ephexin1 can then activate the small GTPase, RhoA, leading to subsequent activation of its effector, Rho-kinase (ROCK), which results in the rearrangement of actin filaments.[30] Through this mechanism, astrocytic processes are able to stabilize individual dendritic protrusions as well as their maturation into spines via ephrin/EphA signaling. Forward signaling involving the activation of EphA4 results in the stabilization of synaptic proteins at the neuromuscular junction.[30] As in the EphA4/ephrinA3-mediated neuron–glia interaction, this process regulates dynamics of the actin cytoskeleton by activating ROCK through ephexin.[30]
Ephrin B/EphB signaling is also involved in synaptic stabilization through different mechanisms. These molecules contain cytoplasmic tails which interact with scaffolding proteins via their PDZ domains to stabilize newly formed CNS synapses.[28] For example, Ephrin B3 interacts with the adaptor protein glutamate-receptor-interacting protein 1 (GRIP-1) to regulate the development of excitatory dendritic shaft synapses.[28] This process, which was identified in cultures of hippocampal neurons, revealed that Eph/ephrin B3 reverse signaling recruits GRIP1 to the membrane of the postsynaptic shaft.[31] Once at the membrane shaft, GRIP1 helps anchor glutamate receptors below the presynaptic terminal. This process also involves the phosphorylation of a serine residue near the ephrin-B carboxyl terminus (proximal to the PDZ-binding motif) that leads to the stabilization of AMPA receptors at synapses.[27]
Another mechanism, found in hippocampal neurons, revealed that EphB signaling could promote spine maturation by modulating Rho GTPase activity, as observed with EphAs.[32] Unlike EphAs, however, the EphB2 receptor has been shown to interact with the postsynaptic N-methyl-D-aspartate receptors (NMDARs) to recruit the GEF Tiam1 to the complex upon ephrinB binding.[32][30][33] Phosphorylation of Tiam1 occurs in response to NMDAR activity, which allows for the influx of calcium that activates Tiam1. This mechanism also results in the modulation of the actin cytoskeleton. As a result of this stabilization, both EphB2 forward signaling and ephrin-B3 reverse signaling has been found to induce LTP via NMDARs.[34]
References
- ↑ Rutishauser U, Jessell TM (July 1988). "Cell adhesion molecules in vertebrate neural development". Physiological Reviews. 68 (3): 819–57. doi:10.1152/physrev.1988.68.3.819. PMID 3293093.
- ↑ "Gerald M. Edelman biography". Nobelprize.org. Retrieved 13 March 2018.
- ↑ Benson DL, Schnapp LM, Shapiro L, Huntley GW (November 2000). "Making memories stick: cell-adhesion molecules in synaptic plasticity". Trends in Cell Biology. 10 (11): 473–82. doi:10.1016/S0962-8924(00)01838-9. PMID 11050419.
- ↑ Bukalo, Olena; Dityatev, Alexander (27 December 2012). "Synaptic Cell Adhesion Molecules". Synaptic Plasticity. Advances in Experimental Medicine and Biology. Vol. 970. Vienna: Springer, Vienna. pp. 97–128. doi:10.1007/978-3-7091-0932-8_5. ISBN 978-3-7091-0932-8. PMID 22351053.
- ↑ Biederer, Thomas; Missler, Markus; Südhof, Thomas (2012). "Synaptic Cell Adhesion". Cold Spring Harbor Perspectives in Biology. Cold Spring Harbor Laboratory Press. 4 (4): a005694. doi:10.1101/cshperspect.a005694. PMC 3312681. PMID 22278667. Retrieved 12 March 2018.
- ↑ Washbourne, Philip; Dityatev, Alexander; Scheiffele, Peter; Biederer, Thomas; Weiner, Joshua A.; Christopherson, Karen S.; El-Husseini, Alaa (20 October 2004). "Cell Adhesion Molecules in Synapse Formation". Journal of Neuroscience. 24 (42): 9244–9249. doi:10.1523/JNEUROSCI.3339-04.2004. PMC 6730099. PMID 15496659.
- ↑ Dalva, Matthew; McClelland, Andrew; Kayser, Matthew (14 February 2007). "Cell adhesion molecules: signalling functions at the synapse". Nature. 8 (3): 206–220. doi:10.1038/nrn2075. PMC 4756920. PMID 17299456.
- 1 2 Bamji SX (July 2005). "Cadherins: actin with the cytoskeleton to form synapses". Neuron. 47 (2): 175–8. doi:10.1016/j.neuron.2005.06.024. PMID 16039559.
- 1 2 3 4 5 6 7 Arikkath J, Reichardt LF (September 2008). "Cadherins and catenins at synapses: roles in synaptogenesis and synaptic plasticity". Trends in Neurosciences. 31 (9): 487–94. doi:10.1016/j.tins.2008.07.001. PMC 2623250. PMID 18684518.
- 1 2 Seong E, Yuan L, Arikkath J (April 2015). "Cadherins and catenins in dendrite and synapse morphogenesis". Cell Adhesion & Migration. 9 (3): 202–13. doi:10.4161/19336918.2014.994919. PMC 4594442. PMID 25914083.
- 1 2 Whalley K (October 2015). "Neural development: a complex competition for spines". Nature Reviews. Neuroscience. 16 (10): 577. doi:10.1038/nrn4024. PMID 26307326.
- 1 2 Bian WJ, Miao WY, He SJ, Qiu Z, Yu X (August 2015). "Coordinated Spine Pruning and Maturation Mediated by Inter-Spine Competition for Cadherin/Catenin Complexes". Cell. 162 (4): 808–22. doi:10.1016/j.cell.2015.07.018. PMID 26255771.
- ↑ Sanes D (January 25, 2011). Development of the Nervous System (3rd ed.). Elsevier. ISBN 978-0-08-092320-8.
- 1 2 Irie K, Shimizu K, Sakisaka T, Ikeda W, Takai Y (December 2004). "Roles and modes of action of nectins in cell-cell adhesion". Seminars in Cell & Developmental Biology. 15 (6): 643–56. doi:10.1016/s1084-9521(04)00088-6. PMID 15561584.
- ↑ Rikitake Y, Mandai K, Takai Y (August 2012). "The role of nectins in different types of cell-cell adhesion". Journal of Cell Science. 125 (Pt 16): 3713–22. doi:10.1242/jcs.099572. PMID 23027581.
- ↑ Takai Y, Shimizu K, Ohtsuka T (October 2003). "The roles of cadherins and nectins in interneuronal synapse formation". Current Opinion in Neurobiology. 13 (5): 520–6. doi:10.1016/j.conb.2003.09.003. PMID 14630213. S2CID 10053035.
- ↑ Craig AM, Kang Y (February 2007). "Neurexin-neuroligin signaling in synapse development". Current Opinion in Neurobiology. 17 (1): 43–52. doi:10.1016/j.conb.2007.01.011. PMC 2820508. PMID 17275284.
- ↑ Dean C, Dresbach T (January 2006). "Neuroligins and neurexins: linking cell adhesion, synapse formation and cognitive function". Trends in Neurosciences. 29 (1): 21–9. doi:10.1016/j.tins.2005.11.003. PMID 16337696. S2CID 11664697.
- ↑ Nam CI, Chen L (April 2005). "Postsynaptic assembly induced by neurexin-neuroligin interaction and neurotransmitter". Proceedings of the National Academy of Sciences of the United States of America. 102 (17): 6137–42. Bibcode:2005PNAS..102.6137N. doi:10.1073/pnas.0502038102. PMC 1087954. PMID 15837930.
- ↑ Brady ST, Siegel GJ, Albers RW, Price DL (2012). Basic neurochemistry : principles of molecular, cellular, and medical neurobiology (Eighth ed.). Waltham, Massachusetts. ISBN 978-0-12-374947-5. OCLC 754167839.
{{cite book}}
: CS1 maint: location missing publisher (link) - 1 2 Missler M, Südhof TC, Biederer T (April 2012). "Synaptic cell adhesion". Cold Spring Harbor Perspectives in Biology. 4 (4): a005694. doi:10.1101/cshperspect.a005694. PMC 3312681. PMID 22278667.
- ↑ Hortsch M (2009). "A Short History of the Synapse – Golgi Versus Ramón y Cajal". In Hortsch M, Umemori H (eds.). The Sticky Synapse. Springer, New York, NY. pp. 1–9. doi:10.1007/978-0-387-92708-4_1. ISBN 978-0-387-92707-7.
- 1 2 Missler M, Zhang W, Rohlmann A, Kattenstroth G, Hammer RE, Gottmann K, Südhof TC (June 2003). "Alpha-neurexins couple Ca2+ channels to synaptic vesicle exocytosis". Nature. 423 (6943): 939–48. Bibcode:2003Natur.423..939M. doi:10.1038/nature01755. PMID 12827191. S2CID 10315093.
- 1 2 Squire LR (2009). Encyclopedia of neuroscience. Amsterdam: Academic Press. ISBN 978-0-08-096393-8. OCLC 503584095.
- ↑ Zhang C, Atasoy D, Araç D, Yang X, Fucillo MV, Robison AJ, Ko J, Brunger AT, Südhof TC (May 2010). "Neurexins physically and functionally interact with GABA(A) receptors". Neuron. 66 (3): 403–16. doi:10.1016/j.neuron.2010.04.008. PMC 3243752. PMID 20471353.
- ↑ Hata Y, Davletov B, Petrenko AG, Jahn R, Südhof TC (February 1993). "Interaction of synaptotagmin with the cytoplasmic domains of neurexins". Neuron. 10 (2): 307–15. doi:10.1016/0896-6273(93)90320-Q. PMID 8439414. S2CID 12954601.
- 1 2 Lisabeth EM, Falivelli G, Pasquale EB (September 2013). "Eph receptor signaling and ephrins". Cold Spring Harbor Perspectives in Biology. 5 (9): a009159. doi:10.1101/cshperspect.a009159. PMC 3753714. PMID 24003208.
- 1 2 3 Bianchi L (2018). Developmental Neurobiology. New York, NY: Garland Science. pp. 299–302. ISBN 9780815344827.
- ↑ Bolton MM, Eroglu C (October 2009). "Look who is weaving the neural web: glial control of synapse formation". Current Opinion in Neurobiology. 19 (5): 491–7. doi:10.1016/j.conb.2009.09.007. PMID 19879129. S2CID 44625935.
- 1 2 3 4 5 Rubenstein J (May 2013). Cellular Migration and Formation of Neuronal Connections: Comprehensive Developmental Neuroscience. San Diego, CA: Elsevier Science & Technology. pp. 659–669. ISBN 978-0-12-397266-8.
- ↑ Flannery DB (September 1988). "Nondisjunction in Down syndrome". American Journal of Medical Genetics. 31 (1): 181–2. doi:10.1002/ajmg.1320310123. PMID 2975924.
- 1 2 Lerner AM (October 1990). "Viral myocarditis as an incidental discovery". Hospital Practice. 25 (10): 81–4, 87–90. doi:10.1016/j.brainres.2006.11.033. PMC 2170431. PMID 2170431.
- ↑ Arvanitis D, Davy A (February 2008). "Eph/ephrin signaling: networks". Genes & Development. 22 (4): 416–29. doi:10.1101/gad.1630408. PMC 2731651. PMID 18281458.
- ↑ Lundgren A, Tibbling L, Henriksson NG (March 2018). "DC-determined displacement of the nystagmus beat in rotatory tests". Practica Oto-Rhino-Laryngologica. 31 (1): 54–64. doi:10.3892/etm.2018.5702. PMC 5795627. PMID 5795627.