火星矿石资源
火星上可能蕴藏有对未来定居者非常有用的矿石[1][2],丰富的火山地貌和遍布的撞击坑是多种矿石的有力证据[3]。虽然在火星上尚未找到任何值得花高昂成本运回地球的东西,但未来定居者从火星上获得的矿石越多,也就越容易在那里建立栖息地[4]。
矿床的形成
矿床是在大量热量的帮助下形成的,在火星上,热量可能来自地下移动的熔岩和陨石撞击。地下液态岩石称为岩浆,当岩浆进入地下洞室,在历经数千年缓慢的冷却中,较重的元素会下沉,包括铜、铬、铁和镍等元素会沉积在底部[5]。岩浆炽热时,许多元素都可自由移动,但随着冷却的开始,这些元素则相互结合形成化合物或矿物。由于有些元素不容易结合成矿物,所以当几乎所有其他元素都结合成化合物或矿物后,它们仍单独存在,这些剩下的元素称为不兼容元素[6],其中一些对人类非常有用,如铌,一种用于制造超导体和特种钢的金属,以及用于电视显示器和节能发光二极管灯泡的镧、钕和铕等[7]。在岩浆团冷却并大部分冻结或结晶成固体后,会残留少量液态岩石,这种液体中含有铅、银、锡、铋和锑等重要物质[8]。有时岩浆室中的矿物非常炽热,以致处于气体状态,另一些则与液体溶液中的水和硫混合。气体和富含矿物质的溶液最终进入裂缝,成为有用的矿脉。矿石矿物,包括不相容元素,在热溶液中保持溶解状态,当溶液冷却时则结晶出来[9],由这些热溶液形成的沉积物称为热液矿床,世界上一些最重要的金、银、铅、汞、锌和钨等矿床就是以这种方式形成的[10][11][12]。南达科他州北部布拉克山上几乎所有的矿山都形成于热液中的矿物沉积[13]。当大量岩浆冷却时,通常会产生裂缝,因为岩浆冷却时会收缩硬化。裂缝出现在冻结的岩浆体和周围岩石中,因此矿石可沉积在附近任何一种岩石中,但矿石矿物必须先通过熔融岩浆体来浓缩[14]。
路易斯安那州立大学进行的研究发现,埃律西昂火山周围有不同类型的火山物质,表明火星可能有岩浆演化,这就有可能为火星上未来的人类找到有用的矿物[15][16]。
火星上的熔岩
火星上有许多巨大的火山,表明过去大片区域都非常炎热。奥林帕斯山是太阳系中最大的火山,而其它较小火山中的一座—什洛尼尔斯火山丘高度也接近地球上的珠穆朗玛峰。
- 显示奥林帕斯山地区有数座大型火山
- 热辐射成像系统显示的熔岩流,注意边缘的形状。
有强力证据表明,火星上的热源普遍以岩脉的形式出现,这表明岩浆在地下流动,像墙壁一样穿过岩层[17]。在某些情况下,火星上的岩脉因侵蚀而暴露出来。
- HiWish计划下高分辨率成像科学设备显示的阿拉伯高地的岩脉,这些笔直的特征可能表明未来定居者在那里能找到有价值的矿床,比例尺为500米。
- HiWish计划下高分辨率成像科学设备显示的陶玛西亚区可能的岩脉,岩脉中可能沉积了价值的矿物。
- HiWish计划下高分辨率成像科学设备显示的奥克夏沼区可能的岩脉。
- HiWish计划下高分辨率成像科学设备显示的可能岩脉,图像位于雅庇吉亚区。
- 热辐射成像系统显示的惠更斯陨击坑附近的岩脉,显示为一条从左上往右下延伸的狭窄暗线。
- HiWish计划下高分辨率成像科学设备显示的岩脉,图像拍摄于卡西乌斯区尼罗瑟提斯地区。
- 科罗拉多州西班牙峰附近的岩脉,像此类岩脉在火星上很常见。
火星大片区域分布着被称为“堑沟”的凹槽,地质学家将其归类为地堑,它们从火山向外绵延数千英里[18]。据信,岩脉有助于地堑的形成[19][20][21]。很多,也许大部分地堑下面都有岩脉。人们可能会在火星上发现岩脉和其他火成岩侵入体,因为地质学家认为,在地下移动的液态岩石数量远超过我们在顶部所看到的火山和熔岩流[22]。
在地球上,辽阔的火山地貌被称为大型火成岩区域(LIPs),这些地方是镍、铜、钛、铁、铂、钯和铬的矿源地[4][23]。火星塔尔西斯地区,包含了一群巨大的火山,被认为是一处大型火成岩区域。
- 高分辨率成像科学设备显示的门农尼亚堑沟群中的地堑,该地堑被认为是由岩浆岩脉而非区域构造拉伸所造成(比例尺为1公里)。
- 高分辨率成像科学设备显示的埃律西昂区刻耳柏洛斯堑沟群。
撞击产生的热量
除了熔岩产生的热量外,当小行星撞击火星表面形成巨大陨石坑时,会产生出大量的热量,大型撞击周围的区域可能需要成百上千年时间才能冷却下来[4]。
在此期间,地下冰会融化、加热、溶解矿物质,然后将它们沉积在撞击产生的裂缝或断层中。对地球的研究记录了产生的裂缝以及填塞在裂缝中的次生矿脉[24][25][26][27]。从环火星飞行卫星拍摄的图像中检测到了撞击坑附近的裂缝[28];机遇号探测车在奋斗撞击坑边缘发现了水相和低度热蚀变区[29],这些区域靠近可让深层流体循环的节理和裂缝附近,从而导致岩石产生化学和热蚀变。因此,火星陨石坑周围区域可能含有多样由撞击间接产生的矿物质[30]。
撞击产生的热量来自几个过程,撞击后,地面会立即反弹,导致较热的岩石被抬升到地表,但,大部分的热量是来自撞击过程产生的动能。这种巨大的热量在早期会产生出一些有用的矿品,然后继续保持一段时间,它们被称为“后生矿床”。撞击裂缝中,富含矿物质的热流体循环导致热液作用,加拿大萨德伯里火成杂岩体中的铜镍硫化物就是一则重要的事例。多年来,萨德伯里地区的矿石每年产生 20 亿美元的价值,萨德伯里地层为我们提供了锌、铜、金和铅等矿石[25][31]。
一组研究奥基陨击坑的科研人员报告了热液作用的有力证据,该陨石坑中的脊线可能是在撞击形成裂缝后产生的。他们利用火星勘测轨道飞行器上的仪器,发现了在地球上通常由撞击引起的热液系统中所见到的蒙脱石、二氧化硅、沸石、蛇纹石、碳酸盐和绿泥石等矿石[32][33][34][35][36][37],其他研究火星陨石坑的科学家则提供了火星上撞后击形成的热液系统证据[38][39][40]。
- 背景相机显示的奥基陨击坑广角视图
- 高分辨率成像科学设备显示的奥基陨击坑中部,箭头指示了脊线位置,沙丘出现在图像顶部附近。
- 上一幅高分辨率成像科学设备图像中的脊线近景图,箭头指示了一处“X”形脊线。
- 显示了带指示脊线箭头的奥基陨击坑中央部分近景,前一幅图像的放大版。
火星表面有大量证据表明,过去湿润的气候,在今天冻结为地下冰;因而,很有可能通过撞击加热建立了热液体系。美国宇航局的火星奥德赛号从轨道上使用伽马射线光谱仪实际测量了冰的分布[41]。在过去,大量的水可能在裂缝中循环并沉积出了新的矿物,在来自火星的陨石中发现了这种称为热液蚀变的作用。2011年2月发表的一项研究,详细介绍了在一颗火星辉橄无球粒陨石矿脉中发现的粘土矿物、蛇纹石和碳酸盐[42][43]。凤凰号着陆器降落时的火箭引擎喷流实际暴露了一层冰,并看到了冰的融化(冰升华后消失)[44][45].
由于地球上大约180座陨石坑中有30%含有矿物或石油和天然气,因此陨石坑似乎促进了自然资源的演变[46]。在地球上与撞击有关作用产生的矿石包括铁、铀、金、铜和镍矿等。 据估计,仅在北美,每年从撞击结构中开采的矿物价值就高达50亿美元[25]。
- 希腊盆地区地形图,这是需要数千年才能冷却的撞击之一。在该地区冷却过程中,可能沉积了大量的矿物。
- 阿耳古瑞盆地地形图,阿耳古瑞区的主要特征,这座巨大的撞击坑也需要数千年的时间才能冷却。
可用材料的直接证据
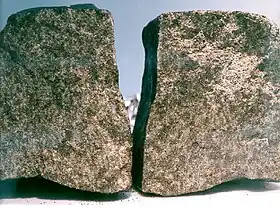
一段时间以来,科学界已认可了一群来自火星陨石,因此,它们代表了该行星的实际样本,并已通过可用的最佳设备在地球上进行了分析。在这些被称为SNC的陨石中,检测到了许多重要元素,镁、铝、钛、铁和铬等在其中较为常见。此外,还发现了微量的锂、钴、镍、铜、锌、铌、钼、镧、铕、钨和金。很有可能在某些地方,这些矿物的密集程度足以进行经济开采[47]。
火星着陆器海盗1号、海盗2号、探路者路、机遇号探测车和勇气号探测车在火星土壤中发现了铝、铁、镁和钛[48]。机遇号发现了一种名为“蓝莓石”的赤铁矿球核,这是一种主要的铁矿石[49],这些蓝莓石很容易被收集起来并还原成用于炼钢的金属铁。
此外,“勇气号”和“机遇号”探测车都在火星表面发现了镍铁陨石[50][51],它们也可用来制造钢铁[52]。
2011年12月,机遇号探测车发现了一道从土壤中突出的石膏矿脉,测试证实它含有钙、硫和水.石膏矿物与探测数据最相符,它可能是由富含矿物质的水穿过岩石裂缝时所形成,这条名为“霍姆斯特克”矿脉位于火星子午线高原中,富含硫酸盐的沉积基岩与奋斗撞击坑边缘裸露的更老火山基岩的交汇区[53]。
- 热盾岩—在另一颗行星上发现的第一颗陨石,它含有93%的铁。
- 海盗号登陆器拍摄了这张火星表面照片并分析了土壤。
黑色沙丘在火星表面很常见,它们的黑色调是由一种叫做玄武岩的火山岩造所成。玄武岩沙丘被认为含有铬铁矿、磁铁矿和钛铁矿等矿物[54]。由于风将它们聚集在一起,因此,它们甚至不必开采,直接挖取就可[55],这些矿物可为未来定居者提供铬、铁和钛等材料。
- 构成挪亚区中一块暗斑的黑色沙丘(可能是玄武岩),火星全球探勘者号拍摄。
- 高分辨率成像科学设备拍摄的挪亚区沙丘全景图
- 高分辨率成像科学设备拍摄的上图中的沙丘特写,注意一些巨石上几乎没有覆盖沙子。
未来火星矿石勘探
从理论上讲,火星上存在矿石资源[55],灵敏的探测设备可探知在何处找到它们,比如陨石坑周围和火山附近。随着拍摄图像的增多,收集到的信息也将越益丰富,这将有助于更好地绘制较小结构的位置,如表明侵入(地表下)火成活动的岩脉。之后,载有重力和磁性测量装置的无人飞行器将能地确定矿床的确切位置。
参考文献
- Cordell, B. 1984. A Preliminary Assessment of Martian Natural Resource Potential. The Case For Mars II.
- Clark, B. 1984. Chemistry of the Martian Surface: Resources for the Manned Exploration of Mars, in The Case For Mars. P. Boston, ed. American Astronautical Society. Univelt Inc. San Diego, CA
- West, M., J. Clarke. 2010. Potential martian mineral resources: Mechanisms and terrestrial analogs. Planetary and Space Science 58, 574–582.ResearchGate (页面存档备份,存于)
- Larry O'Hanlon. . Discovery News. February 22, 2010 [2021-10-27]. (原始内容存档于2012-10-22).
- Namowitx, S. and D. Stone. 1975. Earth Science: The World We Live In. American Book Company. NY, NY.
- (PDF). home.wlu.edu. (原始内容 (PDF)存档于2016-06-11).
- Hsu, Jeremy. . Live Science. June 14, 2010.
- Sorrell, C. 1973. Rocks and Minerals. Golden Press. NY, NY.
- Patterson, Megan Elizabeth. . sierra.sitehost.iu.edu. 2003 [2021-08-02]. (原始内容存档于2021-02-24).
- . Nevada Outback Gems. [2021-10-27]. (原始内容存档于2021-08-27).
- Laimin, Zhu. . Chinese Journal of Geochemistry. 1998, 17 (4): 362–371. S2CID 130661251. doi:10.1007/bf02837988.
- . www.mirandagold.com. [2021-10-27]. (原始内容存档于2013-03-27).
- Gries, John Paul. . 1996. ISBN 0-87842-338-9.
- Pirajno, F. 2004. Metallogeny in the Capricorn Orogen, Western Australia, the result of multiple ore-forming processes. Precambrian Research: 128. 411-439
- David Susko, Suniti Karunatillake, Gayantha Kodikara, J. R. Skok, James Wray, Jennifer Heldmann, Agnes Cousin, Taylor Judice. "A record of igneous evolution in Elysium, a major martian volcanic province. Scientific Reports, 2017; 7: 43177 doi:10.1038/srep43177
- . ScienceDaily. February 24, 2017 [31 July 2021]. (原始内容存档于2021-10-29).
- . MantlePlumes.org. [2021-10-27]. (原始内容存档于2021-02-24).
- Head, J. et al. 2006. The Huygens-Hellas giant dike system on Mars: Implications for Late Noachian-Early Hesperian volcanic resurfacing and climate evolution. Geology: 34. 285-288.
- Goudy, C. and R. Schultz. 2005. Dike intrusions beneath grabens south of Arsia Mons, Mars. Geophysical Research Letters: 32. L05201
- Mege, D. et al. 2003. Volcanic rifting at Martian grabens. Journal of Geophysical Research: 108.
- Wilson, L. and J. Head. 2002. Tharsis-radial graben systems as the surface manifestation of plume-related dike intrusion complexes: Models and implications. Journal of Geophysical Research: 107.
- Crisp, J. 1984. Rates of magma emplacement and volcanic output. J. Volcano. Geotherm. Res: 20. 177-211.
- Ernst, R. 2007. large Igneous Provinces in Canada Through Time and Their Metallogenic Potential. Mineral Deposits of Canada: A Synthesis of Major Deposit-Types, District Metallogeny, the Evolution of Geological Provinces, and Exploration Methods: Geological Association of Canada, Mineral Division, Special Publication No. 5. 929-937.
- Grieve, Richard; Masaitis, V. L. . International Geology Review. 1994, 36 (2): 105–151. doi:10.1080/00206819409465452.
- Grieve, R., V. Masaitis. 1994. The Economic Potential of Terrestrial Impact Craters. International Geology Review: 36, 105-151.
- Osinski, G, J. Spray, and P. Lee. 2001. Impact-induced hydrothermal activity within the Haughton impact structure, Arctic Canada: Generation of a transient, warm, wet oasis. Meteoritics & Planetary Science: 36. 731-745
- Pirajno, F. 2000. Ore Deposits and Mantle Plumes. Kluwer Academic Publishers. Dordrecht, The Netherlands
- Head, J. and J. Mustard. 2006. Breccia Dikes and Crater-Related Faults in Impact Craters on Mars: Erosion and Exposure on the Floor of a 75-km Diameter Crater at the Dichotomy Boundary. Special Issue on Role of Volatiles and Atmospheres on Martian Impact Craters Meteoritics & Planetary Science
- Arvidson, R., et al. 2015. Recent Results from the Opportunity Rover's Exploration of Endeavour Crater, Mars. 46th Lunar and Planetary Science Conference. 1118.pdf
- Crumpler, L., R. Arvidson, W. Farrand, M. Golombek, J. Grant, D. Ming, D. Mittlefehldt, T. Parker. 2015. Opportunity In Situ Geologic Context of Aqueous Alteration Along Offsets in the Rim of Endeavour Crater. 46th Lunar and Planetary Science Conference. 2209.pdf
- Grieve R., A. Therriault. 2000 Vredefort, Sudbury, Chicxulub: Three of a kind? Annual Review of Earth and Planetary Sciences 28: 305-338 Grieve
- Carrozzo, F. et al. 2017. Geology and mineralogy of the Auki Crater, Tyrrhena Terra, Mars: A possible post-impact-induced hydrothermal system. 281: 228-239
- Loizeau, D. et al. 2012. Characterization of hydrated silicate-bearing outcrops in Tyrrhena Terra, Mars: implications to the alteration history of Mars. Icarus: 219, 476-497.
- Naumov, M. 2005. Principal features of impact-generated hydrothermal circulation systems: mineralogical and geochemical evidence. Geofluids: 5, 165-184.
- Ehlmann, B., et al. 2011. Evidence for low-grade metamorphism, hydrothermal alteration, and diagenesis on Mars from phyllosilicate mineral assemblages. Clays Clay Miner: 59, 359-377.
- Osinski, G. et al. 2013. Impact-generated hydrothermal systems on Earth and Mars. Icarus: 224, 347-363.
- Schwenzer, S., D. Kring. 2013. Alteration minerals in impact-generated hydrothermal systems – Exploring host rock variability. Icarus: 226, 487-496.
- Marzo, G., et al. 2010. Evidence for Hesperian impact-induced hydrothermalism on Mars. Icarus: 667-683.
- Mangold, N., et al. 2012. Hydrothermal alteration in a late Hesperian impact crater on Mars. 43rd Lunar and Planetary Science. #1209.
- Tornabene, L., et al. 2009. Parautochthonous megabreccias and possible evidence of impact-induced hydrothermal alteration in Holden crater, Mars. 40th LPSC. #1766.
- . NASA. May 28, 2002 [2021-10-27]. (原始内容存档于2020-02-24).
- . www.spaceref.com.
- H. G. Changela and J. C. Bridges. Alteration assemblages in the nakhlites: Variation with depth on Mars. Meteoritics & Planetary Science, 2011 45(12):1847-1867 doi:10.1111/j.1945-5100.2010.01123.x
- Rayl, A. J. S. . The Planetary Society. Planetary Society. 2008-06-21 [2008-06-23]. (原始内容存档于June 27, 2008).
- . www.nasa.gov. [2021-10-27]. (原始内容存档于2008-07-01).
- . www.spaceref.com.
- Hugh H. Kieffer. . University of Arizona Press. 1992 [7 March 2011]. ISBN 978-0-8165-1257-7. (原始内容存档于2017-03-12).
- Fairen, A. et al. 2009. Nature: 459. 401-404.
- Squyres, et al. 2004. The Opportunity Rover's Athena science investigation at Meridiani Planum. Science: 306. 1598-1703.
- Rodionov, D. et al. 2005. An iron-nickel meteorite on Meridiani Planum: observations by MER Opportunity's Mossbauer Spectrometer, European Geosciences Union in Geophysical Research Abstracts: 7. 10242
- Yen, A., et al. Nickel on Mars: constraints on meteoritic material at the surface. Journal of Geophysical Research-Planets: 111. E12S11
- Landis, G. 2009. Meteoritic steel as a construction resource on Mars. Acta Astronautica: 64. 183-187.
- . www.nasa.gov. [2021-10-27]. (原始内容存档于2017-06-25).
- Ruzicka, G. et al. 2001. Comparative geochemistry of basalts from the Moon, Earth, HED asteroid, and Mars: implications for the origin of the Moon. Geochimica et Cosmochimica ACTA: 65. 979-997.
- West, M. and J. Clarke. 2010. Potential martian mineral resources: Mechanisms and terrestrial analogs. Planetary and Space Science: 58. 574-582. ResearchGate (页面存档备份,存于)