The androgen backdoor pathway is a collective name for all metabolic pathways where physiologically significant androgens are synthesized from 21-carbon (C
21) steroids (pregnanes) by their 5α-reduction, bypassing testosterone and/or androstenedione.
A backdoor pathway is an alternative to the conventional, canonical androgenic pathway that involves testosterone and/or androstenedione. In the canonical pathway, 5α-dihydrotestosterone is synthesized directly from testosterone through the action of the enzyme 5α-reductase in tissues where 5α-reductase enzymes are highly expressed, such as the prostate gland, hair follicles, and skin. In both pathways (canonical and backdoor), the enzyme 5α-reductase plays a crucial role. However, in the androgen backdoor pathway, 5α-reductase acts on the C
21 steroids (pregnanes), leading to the reduction of the 4,5-double bond in these C
21 steroids, starting a long chain of transformations to 5α-dihydrotestosterone, whereas in a canonical pathway 5α-reductase acts on the 4,5-double bond in testosterone to produce 5α-dihydrotestosterone directly.
Initially described as a pathway where 5α-reduction of 17α-hydroxyprogesterone ultimately leads to 5α-dihydrotestosterone, several other pathways have been since then discovered that lead to 11-oxygenated androgens which are also clinically significant androgens.
The role of these backdoor pathways relates to early male sexual differentiation, hair growth patterns, regulation of secretory functions, sebum production by skin cells, and various biological processes where androgens are involved. Understanding these processes is essential for developing effective treatments for conditions associated with hyperandrogenism. In individuals with congenital adrenal hyperplasia (CAH) due to enzyme deficiencies like 21-hydroxylase deficiency or cytochrome P450 oxidoreductase deficiency (POR), activation of these alternate routes can occur at any age when there is an increase in circulating levels of certain precursors such as progesterone or 17α-hydroxyprogesterone, and can lead to symptoms of hyperandrogenism, such as acne, hirsutism (growth of hair where they should not normally grow), polycystic ovary syndrome or prostate gland enlargement.
Function
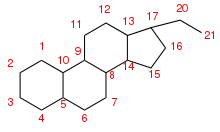
The androgen backdoor pathways are vital for creating androgens from 21-carbon (C
21) steroids, known as pregnanes. A 21-carbon steroid is a steroid molecule with 21 carbon atoms,[2] hence, their chemical formula contains C
21. For example, the chemical formula of progesterone is C
21H
30O
2. That's why 21-carbon steroids are denoted as C
21-steroids, 19-carbon steroids are denoted as C
19 steroids, and so on.[3][4]
The androgen backdoor pathways occur without the involvement of testosterone (T) and/or androstenedione (A4), which are part of the conventional, canonical androgenic pathway.[5][6]
The androgen backdoor pathways are characterized by 5α-reduction of C
21 steroids. The 5α-reduction is a chemical reaction where a functional group attached to the carbon in position 5α of the steroid nucleus is reduced, and a double bond between carbon atoms numbered 4 and 5 (see § Figure 1) in the steroid molecule is replaced to the single bond in a chemical reaction catalyzed by the SRD5A1 enzyme (see examples on the § Figure 2 denoted by arrows marked by "SRD5A1" in the square box).[1]
In the early 2000s, the 5α-reduction of 17α-hydroxyprogesterone was described in medical literature as the start of the backdoor pathway to DHT biosynthesis. This pathway was first described in the marsupials and later confirmed in humans.[7] That's why the backdoor pathway of DHT biosynthesis from 17OHP can be called a marsupial pathway.[8] This pathway is also present in other mammals,[9][10] such as rats, and are studied in the other mammals as a way to better understand these pathways in humans.[2][9][11]
Sexual differentiation is a process by which hormones determine anatomic phenotype, mainly the development of the reproductive organs.[8]
Marsupials, and in particular, tammar wallabies (Notamacropus eugenii)[12] are especially useful for studying the processes of sexual differentiation and development in the context of androgen biosynthesis,[8][13] because sexual differentiation in these species occurs only after birth, with testes beginning to form two days after birth and ovaries only on the eighth day after birth. This feature of post-natal early sexual differentiation allows scholars to study the influence of hormones on the body from the very beginning of the process of sexual differentiation, as well as the pathways of biosynthesis of these hormones. Tammar wallabies are particularly interesting due to the fact that all these hormones, pathways, and the ways in which hormones affect body features and growth of different organs can be studied when the organism is already born, unlike in other mammals such as rats, where sexual differentiation in a fetus occurs inside the placenta before birth.[14][15][16] The discovery of the backdoor pathway to DHT biosynthesis in tammar wallaby pouch young in 2003 has expanded scholars' understanding of androgen production in humans and has contributed to the development of treatments and management of conditions associated with under- and over-production of androgens.[8]
The backdoor pathway to DHT biosynthesis in humans was described in 2004 in[2] as 5α-reduction of 17α-hydroxyprogesterone (17OHP) which is a first step in a pathway that ultimately leads to the production of 5α-dihydrotestosterone (DHT). Over the following two decades, several other distinct pathways have been discovered that lead to the synthesis of 11-oxygenated androgens, which are clinically relevant androgens, i.e., the potent agonists of the androgen receptors.[17]
The androgen response mechanism involves androgens binding to androgen receptors in the cytoplasm, which then move into the nucleus and control gene transcription by interacting with specific DNA regions called androgen responsive elements.[18] This response mechanism plays a crucial role in male sexual differentiation and puberty, as well as other tissue types and processes, such as the prostate gland (regulate secretory functions), hair follicles (androgens influence hair growth patterns), skin (androgens regulate sebum production and the thickening and maturation of the skin), and muscle (contribute to the development and maintenance of muscle mass and strength).[19][20]
Different androgens have different effects on androgen receptors because they have different degrees of binding and activating the receptors. Clinically significant androgens are those androgens that have a strong influence on the development and functioning of male sexual characteristics, unlike clinically insignificant androgens, which have low biological activity or are quickly metabolized into other steroids. Clinically insignificant androgens do not have a notable influence on the development and functioning of male or female sexual characteristics, they can be products of the metabolism of more active androgens, such as testosterone (T), or their precursors.[21]
Importance
The discovery of the backdoor pathway to 5α-dihydrotestosterone (DHT) in the tammar wallaby in the early 2000s[12] opened new avenues for understanding the biosynthesis of androgens in humans, by suggesting the possibility of alternative pathways for androgen synthesis in humans in addition to conventional pathways. This finding observed in tammar wallaby prompted research into identifying and characterizing similar pathways in humans, leading to a better understanding of the regulation, metabolism, and therapeutic targeting of androgen biosynthesis in human health and diseases related to excessive or insufficient androgen biosynthesis when the classical androgen pathway could not fully explain the observed conditions in patients. Subsequently, other backdoor pathways leading to potent 11-oxygenated androgens have also been characterized,[22] providing further insight into the synthesis of androgens in vivo. Understanding these pathways is critical for the development of effective treatments for conditions related to androgen biosynthesis.[23]
Biochemistry
Dihydrotestosterone backdoor biosynthesis
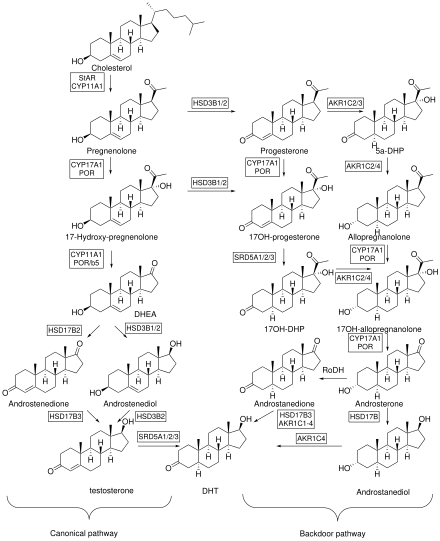
Gene | Enzyme | Pathway | Directional preference | Tissue distribution |
---|---|---|---|---|
AKR1C1 | 3α-HSD4 | Backdoor | Reductive | Liver, testis, lung, breast, uterus, brain |
AKR1C2 | 3α-HSD3 | Backdoor | Reductive | Liver, prostate, lung, uterus, brain |
AKR1C3 | 3α-HSD2 | Backdoor | Reductive | Prostate, breast, liver, adrenal, testis, lung |
AKR1C4 | 3α-HSD1 | Backdoor | Reductive | Liver >> adrenal/gonad |
HSD3B1 | 3β-HSD1 | Backdoor/Canonical | Oxidative | Testis, adrenal, placenta |
HSD3B2 | 3β-HSD2 | Backdoor/Canonical | Oxidative | Testis, adrenal |
HSD17B3 | 17β-HSD3 | Backdoor/Canonical | Oxidative | Leydig cells (testis) |
HSD17B6 | 17β-HSD6 (RoDH) | Backdoor | Oxidative | Prostate |
SRD5A1 | 5α-reductase, type 1 | Backdoor/Canonical | Reductive | Wide tissue expression |
SRD5A2 | 5α-reductase, type 2 | Backdoor/Canonical | Reductive | Prostate |
SRD5A3 | 5α-reductase, type 3 | Backdoor/Canonical | Reductive | Wide tissue expression |
StAR | steroidogenic acute regulatory protein | Backdoor/Canonical | N/A | Adrenal gland and Leydig cells |
CYP11A1 | P450scc | Backdoor/Canonical | Oxidative | Adrenal gland and testis |
CYP17A1 | Steroid 17-alpha-hydroxylase | Backdoor/Canonical | Oxidative | Adrenal gland and testis |
POR | cytochrome b5, P450 oxidoreductase | Backdoor/Canonical | N/A | Liver, lower levels in other tissues |
The primary feature of the androgen backdoor pathway is that 17α-hydroxyprogesterone (17OHP) can be 5α-reduced and finally converted to 5α-dihydrotestosterone (DHT) via an alternative route that bypasses the conventional[24] intermediates androstenedione (A4) and testosterone (T).[2][25]
This route is activated during normal prenatal development and leads to early male sexual differentiation.[26][27][28] 5α-dihydrotestosterone synthesized by this route plays a critical role in the development of male sexual characteristics, including the differentiation and maturation of the male external genitalia, the prostate gland, and other male reproductive structures. By bypassing the conventional intermediates (A4 and T), this pathway ensures the timely and appropriate development of male sexual traits in early embryonic and fetal stages. Both canonical and backdoor pathways are essential in normal male embryonic development.[29][26][30] A disruption in the backdoor pathway can lead to incomplete or altered male sexual differentiation. This disruption may result in abnormalities or underdevelopment of the male external genitalia, prostate gland, and other male reproductive structures. The specific consequences can vary depending on the nature and extent of the disruption and may lead to conditions such as ambiguous genitalia or other disorders of sexual development (DSD), where the individual's physical and sexual characteristics do not align clearly with typical male, i.e., undervirilization of male infants.[29][31][22][8] Undervirilization refers to insufficient development of male characteristics due to below-normal effects of androgens during prenatal development. After birth, it may manifest as markedly underdeveloped male genitalia.[32]
The backdoor pathway of DHT biosynthesis from 17OHP to DHT was first described in the marsupials and later confirmed in humans.[7][8] Both the canonical and backdoor pathways of DHT biosynthesis are required for normal development of male genitalia in humans. As such, defects in the backdoor pathway from 17OHP or progesterone (P4) to DHT lead to undervirilization in male fetuses because placental P4 is the precursor of DHT via the backdoor pathway.[29]
In 21-hydroxylase deficiency[25] or cytochrome P450 oxidoreductase deficiency,[33] this route may be activated regardless of age and sex by even a mild increase in circulating 17OHP levels.[34]
While 5α-reduction is the last transformation in the classical androgen pathway, it is the first step in the backdoor pathways to 5α-dihydrotestosterone that acts on either 17OHP or P4 which are ultimately converted to DHT.[2]
17α-Hydroxyprogesterone pathway
The first step of this pathway is the 5α-reduction of 17OHP to 5α-pregnan-17α-ol-3,20-dione (referred to as 17OHDHP or 17α-hydroxy-dihydroprogesterone). The reaction is catalyzed by SRD5A1.[11][33]
17OHDHP is then converted to 5α-pregnane-3α,17α-diol-20-one (5α-Pdiol) via 3α-reduction by a 3α-hydroxysteroid dehydrogenase isozyme (AKR1C2 and AKR1C4)[31][7] or HSD17B6, that also has 3α-reduction activity.[35][36] 5α-Pdiol is also known as 17α-hydroxyallopregnanolone or 17OH-allopregnanolone.[2]
5α-Pdiol is then converted to 5α-androstan-3α-ol-17-one, also known as androsterone (AST) by 17,20-lyase activity of CYP17A1 which cleaves a side-chain (C17-C20 bond) from the steroid nucleus, converting a 21-C carbon steroid (a pregnane) to C
19 steroid (an androstane or androgen).[2]
AST is 17β-reduced to 5α-androstane-3α,17β-diol (3α-diol) by HSD17B3 or AKR1C3.[37]
The final step is 3α-oxidation of 3α-diol in target tissues to DHT by an enzyme that has 3α-hydroxysteroid oxidase activity, such as AKR1C2,[38] HSD17B6, HSD17B10, RDH16, RDH5, and DHRS9.[33] This oxidation is not required in the classical androgen pathway. The pathway can be summarized as: 17OHP → 17OHDHP → 5α-Pdiol → AST → 3α-diol → DHT.[2]
Progesterone pathway
The pathway from progesterone (P4) to DHT is similar to that described above from 17OHP to DHT, but the initial substrate for 5α-reductase here is P4 rather than 17OHP. Placental P4 in the male fetus is the feedstock, that is, a starting point, the initial substrate, for the backdoor pathway found operating in multiple non-gonadal tissues.[31] The first step in this pathway is 5α-reduction of P4 towards 5α-dihydroprogesterone (5α-DHP) by SRD5A1. 5α-DHP is then converted to allopregnanolone (AlloP5) via 3α-reduction by AKR1C2 or AKR1C4. AlloP5 is then converted to 5α-Pdiol by the 17α-hydroxylase activity of CYP17A1. The pathway then proceeds the same way as the pathway that starts from 17OHP, and can be summarized as: P4 → 5α-DHP → AlloP5 → 5α-Pdiol → AST → 3α-diol → DHT.[2]
11-Oxygenated androgen backdoor biosynthesis
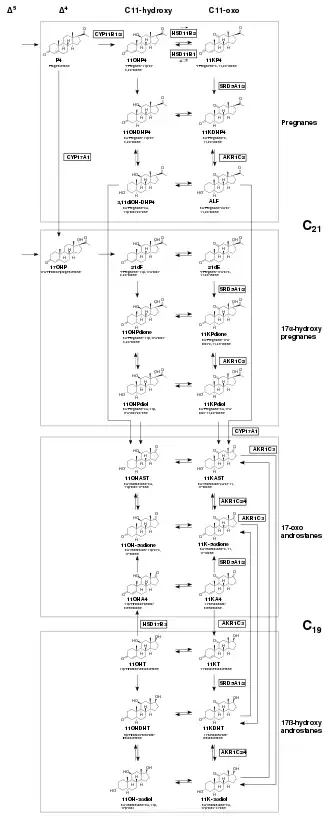
21 steroids (pregnanes) to C
19 steroids (androstanes). Some transformations which are presumed to exist but not yet shown to exist are depicted with dotted arrows. Some CYP17A1 mediated reactions that transform 11-oxygenated androgens classes (grey box) are omitted for clarity. Δ5 compounds that are transformed to Δ4 compounds are also omitted for clarity.[1]
There are two known clinically significant 11-oxygenated androgens, 11-ketotestosterone (11KT) and 11-ketodihydrotestosterone (11KDHT), which both bind and activate the androgen receptor with affinities, potencies, and efficacies that are similar to that of testosterone (T) and DHT, respectively.[17]
As for 11β-hydroxytestosterone (11OHT) and 11β-hydroxydihydrotestosterone (11OHDHT), the androgenicity of these steroids is a point of research. Although some studies[39][40][1] suggest that though 11β-hydroxytestosterone (11OHT) and 11β-hydroxydihydrotestosterone (11OHDHT) may not have significant androgenic activity as they were once thought to possess, they may still be important precursors to androgenic molecules. The relative importance of the androgens depends on their activity, circulating levels and stability. The steroids 11β-hydroxyandrostenedione (11OHA4) and 11-ketoandrostenedione (11KA4) have been established as having minimal androgen activity, but remain important molecules in this context since they act as androgen precursors.[41][42]
The backdoor pathways to 11-oxygenated androgens can be broadly defined as two Δ4 steroid entry points (17OHP and P4) that can undergo a common sequence of three transformations:
Clinical significance
Congenital adrenal hyperplasia
In congenital adrenal hyperplasia (CAH) due to deficiency of 21-hydroxylase[25] or cytochrome P450 oxidoreductase (POR),[33][29] the associated elevated 17OHP levels result in flux through the backdoor pathway to DHT that begins with 5α-reduction of 17OHP. This pathway may be activated regardless of age and sex and cause symptoms of androgen excess[44] In adult females, excess androgens can cause hirsutism (excessive hair growth), alopecia (hair loss), menstrual irregularities, infertility, and polycystic ovary syndrome (PCOS). In adult males, excess androgens can cause prostate enlargement, prostate cancer, and reduced sperm quality. In adults of both sexes, excess androgens can also cause metabolic disturbances, such as insulin resistance, dyslipidemia, hypertension, and cardiovascular disease.[45] In fetus, excess of androgens due to excess of fetal 17OHP in CAH may contribute to DHT synthesis that leads to external genital virilization in newborn girls with CAH.[33] P4 levels may also be elevated in CAH,[46][47] leading to androgen excess via the backdoor pathway from P4 to DHT.[48] 17OHP and P4 may also serve as substrates to 11-oxygenated androgens in CAH.[49]
Masculinization of female external genitalia in a fetus due to the mother's intake of certain exogenous hormones—the so-called progestin-induced virilization—is usually less noticeable than in congenital adrenal hyperplasia (CAH), and unlike CAH, it does not cause progressive virilization.[50]
Serum levels of the C
21 11-oxygenated steroids: 21-deoxycorticosterone (11OHP4) and 21-deoxycortisol (21dF), have been known to be elevated in both non-classical and classical forms of CAH since about 1990,[51][52] and liquid chromatography–mass spectrometry profiles that include these steroids have been proposed for clinical applications,[53] including newborn screening.[54] Classical CAH patients receiving glucocorticoid therapy had C
19 11-oxygenated steroid serum levels that were elevated 3-4 fold compared to healthy controls.[55] In that same study, the levels of C
19 11-oxygenated androgens correlated positively with conventional androgens in women but negatively in men. The levels of 11KT were 4 times higher compared to that of T in women with the condition. In adult women with CAH, the ratio of DHT produced in a backdoor pathway to that produced in a conventional pathway increases as control of androgen excess by glucocorticoid therapy deteriorates.[56] In CAH patients with poor disease control, 11-oxygenated androgens remain elevated for longer than 17OHP, thus serving as a better biomarker for the effectiveness of the disease control.[57][58] In males with CAH, 11-oxygenated androgen levels may indicate the presence of testicular adrenal rest tumors.[58][59]
Fetal development
In order for the male genitalia to develop properly in humans, both the classical and backdoor pathways are essential as means of DHT biosynthesis.[26][29] Deficiencies in the backdoor pathway that converts 17OHP or P4 into DHT can result in undervirilization of the male fetus.[60][61] This underviriliztion may happen because placental P4 acts as an important precursor to fetal DHT specifically within the backdoor pathway that should not be disrupted.[31][7][11]
Undervirilization refers to an incomplete masculinization of the male fetus. It can have consequences such as ambiguous genitalia or underdeveloped reproductive organs including the penis and testes.[62] These conditions may impact fertility, sexual function, and can also affect an individual's overall gender identity later in life.[63]
A case study[7] involving five individuals with a 46,XY (male) chromosomal pattern from two families revealed that their DSD, manifested in unusual genital appearance, was caused by mutations in the AKR1C2 and/or AKR1C4 genes. These genes are exclusively involved in the backdoor pathway of 5α-dihydrotestosterone (DHT) production. Mutations in the AKR1C3 and genes involved in the classical androgen pathway were excluded as the causes for the atypical genital appearance. Interestingly, their female relatives with a 46,XX chromosomal pattern who had the same mutations exhibited normal physical characteristics and fertility. Although both AKR1C2 and AKR1C4 enzymes are needed for DHT synthesis in a backdoor pathway, the study found that mutations in AKR1C2 only were sufficient for disruption.[7] However, these AKR1C2/AKR1C4 variants leading to DSD are rare and have been only so far reported in just those two families.[64] This case study highlights the role of AKR1C2/4 in the alternative androgen pathways.[8]
Isolated 17,20-lyase deficiency syndrome due to variants in CYP17A1, cytochrome b5, and POR may also disrupt the backdoor pathway to DHT, as the 17,20-lyase activity of CYP17A1 is required for both classical and backdoor androgen pathways.[60] This rare deficiency can lead to DSD in both sexes, with affected girls being asymptomatic until puberty, when they show amenorrhea.[64]
11-oxygenated androgens may play important roles in DSDs.[65][66][33] 11-oxygenated androgen fetal biosynthesis may coincide with the key stages of production of cortisol — at weeks 8–9, 13–24, and from 31 and onwards. In these stages, impaired CYP17A1 and CYP21A2 activity lead to increased ACTH due to cortisol deficiency and the accumulation of substrates for CYP11B1 in pathways to 11-oxygenated androgens and could cause abnormal female fetal development.[65][67]
Cancer
The backdoor pathway to DHT plays a role in the development of androgen-sensitive cancers, such as prostate cancer. In some cases, tumor cells have been found to possess higher levels of enzymes involved in this pathway, resulting in increased production of DHT.[68]
Androgen deprivation therapy (ADT) is a common treatment for prostate cancer, which involves reducing the levels of androgens, specifically T and DHT, in the body.[68] This treatment is done through the use of medications that aim to block the production or action of these hormones. While ADT can be effective in slowing the growth of prostate cancer, it also has several drawbacks, one of which is the potential for increased production of P4 and activation of the backdoor pathway of DHT biosynthesis where P4 serves as a substrate. Normally, this pathway is not very active in healthy adult males, as the majority of DHT is produced through the classical pathway, which involves the direct conversion of T into DHT by one of the SRD5A isozymes. However, when T levels are reduced through ADT, the body may compensate by increasing the production of P4, which can then serve as a substrate for the backdoor pathway. One of the main drawbacks of this increased production of P4 leads to an increase in DHT levels, which fuel the growth of prostate cancer cells. This increased production of P4 and DHT can result in the cancer becoming resistant to ADT and continuing to grow and spread. Additionally, the increased levels of P4 can also cause side effects such as weight gain, fatigue, and mood swings (extreme or rapid changes in mood).[68]
In prostate cancer, removal of testicular T through castration (surgical or chemical removal or inactivation of testicles) helps eliminate the growth-promoting effects of androgens.[68] However, in some cases, metastatic tumors can develop into castration-resistant prostate cancer (CRPC).[69] While castration reduces serum T levels by 90-95%, it only decreases DHT in the prostate gland by 50%. This difference between the magnitude of androgen levels confirms that the prostate has enzymes capable of producing DHT even without testicular T.[70] In addition to DHT production within the prostate, researchers found that 11-oxygenated androgens play a role in maintaining total circulating androgen pool levels which are relevant to the amounts of clinically significant androgens in the body.[71] These 11-oxygenated androgens contribute greatly to reactivating androgen signaling in patients with CRPC.[68][37] Serum levels of 11KT, one type of 11-oxygenated androgen, are higher than any other androgen in approximately 97% of CRPC patients. In fact, 11-oxygenated androgens make up around 60% of the total active androgen pool in such patients. Unlike T or DHT, these levels of 11-oxygenated androgens remain unaffected by castration therapy.[68]
Benign prostatic hyperplasia, chronic prostatitis/chronic pelvic pain syndrome
Androgens are known to play a crucial role not only in prostate cancer but also in other prostate-related conditions such as benign prostatic hyperplasia (BPH) and chronic prostatitis/chronic pelvic pain syndrome (CP/CPPS).[70] In BPH, C
21 11-oxygenated steroids (pregnanes) have been identified are precursors to androgens in BHP.[72] Specifically, steroids like 11OHP4 and 11KP4 can be converted to 11KDHT, an 11-oxo form of DHT with the same potency. These precursors have also been detected in tissue biopsy samples from patients with BPH, as well as in their serum levels. The relationship between steroid serum levels and CP/CPPS suggests that deficiencies in the enzyme CYP21A2 may contribute to the development of this condition. Non-classical congenital adrenal hyperplasia (CAH) resulting from CYP21A2 deficiency is typically considered asymptomatic in men. However, non-classical CAH could be a comorbidity associated with CP/CPPS.[73][74][75]
History
In 1987, Eckstein et al.[76] demonstrated that 5α-androstane-3α,17β-diol (3α-diol) is preferentially produced from 17α-hydroxyprogesterone (17OHP). The function of 3α-diol was not known at that time.[2]
In 2000, Shaw et al.[27] demonstrated that circulating 3α-diol mediates prostate development in tammar wallaby pouch young via conversion to DHT in target tissues. Tammar wallaby pouch young do not show sexually dimorphic circulating levels of T and DHT during prostate development which suggests that another androgenization mechanism was responsible. While 3α-diol's androgen receptor binding affinity is five orders of magnitude lower than DHT (generally described as AR inactive), it was known that 3α-diol can be oxidized back to DHT via the action of a number of dehydrogenases.[77]
In 2003, Wilson et al.[12] demonstrated that 5α-reductase expression in this tissue enabled a novel pathway from 17OHP to 3α-diol without T as an intermediate.[12]
In 2004, Mahendroo et al.[28] demonstrated that an overlapping novel pathway is operating in mouse testes, generalizing what had been demonstrated in tammar wallaby.[28]
The term "backdoor pathway" was coined by Auchus in 2004[2] and defined as a route to DHT that: (1) bypasses conventional intermediates androstenedione (A4) and T; (2) involves 5α-reduction of C
21 pregnanes to C
19 androstanes; and (3) involves the 3α-oxidation of 3α-diol to DHT. The backdoor pathway explains how androgens are produced under certain normal and pathological conditions in humans when the classical androgen pathway cannot fully explain the observed consequences.[2]
The clinical relevance of these results published by Auchus in 2004 was demonstrated in 2012 for the first time when Kamrath et al.[25] attributed the urinary metabolites to the androgen backdoor pathway from 17OHP to DHT in patients with steroid 21-hydroxylase (encoded by the gene CYP21A2) enzyme deficiency.[25]
Barnard et al.[22] in 2017 demonstrated metabolic pathways from C
21 steroids to 11KDHT that bypasses A4 and T, an aspect that is similar to that of the backdoor pathway to DHT. These newly discovered pathways to 11-oxygenated androgens were also described as "backdoor" pathways due to this similarity, and were further characterized in subsequent studies.[78][49]
Note
This article was submitted to WikiJournal of Medicine for external academic peer review in 2022 (reviewer reports). The updated content was reintegrated into the Wikipedia page under a CC-BY-SA-3.0 license (2023). The version of record as reviewed is:
Maxim Masiutin, Maneesh Yadav, et al. (3 April 2023). "Alternative androgen pathways" (PDF). WikiJournal of Medicine. 10 (1): 3. doi:10.15347/WJM/2023.003. ISSN 2002-4436. Wikidata Q100737840.
List of figures
See also
References
- 1 2 3 4 Masiutin MG, Yadav MK (2023). "Alternative androgen pathways". WikiJournal of Medicine. 10: X. doi:10.15347/WJM/2023.003. S2CID 257943362.
- 1 2 3 4 5 6 7 8 9 10 11 12 Auchus RJ (November 2004). "The backdoor pathway to dihydrotestosterone". Trends in Endocrinology and Metabolism. 15 (9): 432–438. doi:10.1016/j.tem.2004.09.004. PMID 15519890. S2CID 10631647.
- ↑ "Progesterone". National Library of Medicine.
- ↑ Andrew ER, Jurga K, Radomski JM, Reynhardt EC (July 1992). "Proton relaxation NMR study of polycrystalline progesterone". Solid State Nucl Magn Reson. 1 (3): 121–5. doi:10.1016/0926-2040(92)90012-x. PMID 1365723.
progesterone (4-pregnene-3,20-dione, C21H30O2)
- ↑ Kater CE, Giorgi RB, Costa-Barbosa FA (March 2022). "Classic and current concepts in adrenal steroidogenesis: a reappraisal". Archives of Endocrinology and Metabolism. 66 (1): 77–87. doi:10.20945/2359-3997000000438. PMC 9991025. PMID 35263051.
- ↑ Lawrence BM, O'Donnell L, Smith LB, Rebourcet D (December 2022). "New Insights into Testosterone Biosynthesis: Novel Observations from HSD17B3 Deficient Mice". International Journal of Molecular Sciences. 23 (24): 15555. doi:10.3390/ijms232415555. PMC 9779265. PMID 36555196.
- 1 2 3 4 5 6 Flück CE, Meyer-Böni M, Pandey AV, Kempná P, Miller WL, Schoenle EJ, Biason-Lauber A (August 2011). "Why boys will be boys: two pathways of fetal testicular androgen biosynthesis are needed for male sexual differentiation". American Journal of Human Genetics. 89 (2): 201–218. doi:10.1016/j.ajhg.2011.06.009. PMC 3155178. PMID 21802064.
- 1 2 3 4 5 6 7 8 9 Biason-Lauber A, Pandey AV, Miller WL, Flück CE (January 2014). "Marsupial pathway in humans.". In New MI, Lekarev O, Parsa A, Yuen TT, O'Malley B, Hammer GD (eds.). Genetic Steroid Disorders. Academic Press. pp. 215–224. doi:10.1016/B978-0-12-416006-4.00015-6. ISBN 978-0-12-416006-4.
- 1 2 Renfree MB, Shaw G (September 2023). "The alternate pathway of androgen metabolism and window of sensitivity". J Endocrinol. 258 (3). doi:10.1530/JOE-22-0296. PMID 37343228. S2CID 259222117.
- ↑ Draskau MK, Svingen T (2022). "Azole Fungicides and Their Endocrine Disrupting Properties: Perspectives on Sex Hormone-Dependent Reproductive Development". Front Toxicol. 4: 883254. doi:10.3389/ftox.2022.883254. PMC 9097791. PMID 35573275.
- 1 2 3 Fukami M, Homma K, Hasegawa T, Ogata T (April 2013). "Backdoor pathway for dihydrotestosterone biosynthesis: implications for normal and abnormal human sex development". Developmental Dynamics. 242 (4): 320–329. doi:10.1002/dvdy.23892. PMID 23073980. S2CID 44702659.
- 1 2 3 4 Wilson JD (2003). "5alpha-androstane-3alpha,17beta-diol is formed in tammar wallaby pouch young testes by a pathway involving 5alpha-pregnane-3alpha,17alpha-diol-20-one as a key intermediate". Endocrinology. 144 (2): 575–80. doi:10.1210/en.2002-220721. PMID 12538619. S2CID 84765868.
- ↑ Biason-Lauber A, Miller WL, Pandey AV, Flück CE (May 2013). "Of marsupials and men: "Backdoor" dihydrotestosterone synthesis in male sexual differentiation". Mol Cell Endocrinol. 371 (1–2): 124–32. doi:10.1016/j.mce.2013.01.017. PMID 23376007. S2CID 3102436.
- ↑ Leihy MW, Shaw G, Wilson JD, Renfree MB (July 2004). "Penile development is initiated in the tammar wallaby pouch young during the period when 5alpha-androstane-3alpha,17beta-diol is secreted by the testes". Endocrinology. 145 (7): 3346–52. doi:10.1210/en.2004-0150. PMID 15059957.
- ↑ Glickman SE, Short RV, Renfree MB (November 2005). "Sexual differentiation in three unconventional mammals: spotted hyenas, elephants and tammar wallabies". Horm Behav. 48 (4): 403–17. doi:10.1016/j.yhbeh.2005.07.013. PMID 16197946. S2CID 46344114.
- ↑ Chen Y, Renfree MB (January 2020). "Hormonal and Molecular Regulation of Phallus Differentiation in a Marsupial Tammar Wallaby". Genes (Basel). 11 (1): 106. doi:10.3390/genes11010106. PMC 7017150. PMID 31963388.
- 1 2 Snaterse G, Hofland J, Lapauw B (January 2023). "The role of 11-oxygenated androgens in prostate cancer". Endocr Oncol. 3 (1): e220072. doi:10.1530/EO-22-0072. PMC 10305623. PMID 37434644.
- ↑ Van-Duyne G, Blair IA, Sprenger C, Moiseenkova-Bell V, Plymate S, Penning TM (2023). "The androgen receptor". Vitamins and Hormones. 123: 439–481. doi:10.1016/bs.vh.2023.01.001. ISBN 9780443134555. PMID 37717994.
- ↑ Alemany M (October 2022). "The Roles of Androgens in Humans: Biology, Metabolic Regulation and Health". International Journal of Molecular Sciences. 23 (19): 11952. doi:10.3390/ijms231911952. PMC 9569951. PMID 36233256.
- ↑ Ceruti JM, Leirós GJ, Balañá ME (April 2018). "Androgens and androgen receptor action in skin and hair follicles". Molecular and Cellular Endocrinology. 465: 122–133. doi:10.1016/j.mce.2017.09.009. hdl:11336/88192. PMID 28912032. S2CID 3951518.
- ↑ Cussen L, McDonnell T, Bennett G, Thompson CJ, Sherlock M, O'Reilly MW (August 2022). "Approach to androgen excess in women: Clinical and biochemical insights". Clin Endocrinol (Oxf). 97 (2): 174–186. doi:10.1111/cen.14710. PMC 9541126. PMID 35349173.
- 1 2 3 Barnard L, Gent R, van Rooyen D, Swart AC (November 2017). "Adrenal C11-oxy C
21 steroids contribute to the C11-oxy C
19 steroid pool via the backdoor pathway in the biosynthesis and metabolism of 21-deoxycortisol and 21-deoxycortisone". The Journal of Steroid Biochemistry and Molecular Biology. 174: 86–95. doi:10.1016/j.jsbmb.2017.07.034. PMID 28774496. S2CID 24071400. - ↑ Turcu AF, Auchus RJ (June 2017). "Clinical significance of 11-oxygenated androgens". Curr Opin Endocrinol Diabetes Obes. 24 (3): 252–259. doi:10.1097/MED.0000000000000334. PMC 5819755. PMID 28234803.
- ↑ Kinter KJ, Anekar AA (2021). Biochemistry, Dihydrotestosterone. StatPearls. PMID 32491566. NCBI NBK557634.
- 1 2 3 4 5 Kamrath C, Hochberg Z, Hartmann MF, Remer T, Wudy SA (March 2012). "Increased activation of the alternative "backdoor" pathway in patients with 21-hydroxylase deficiency: evidence from urinary steroid hormone analysis". The Journal of Clinical Endocrinology and Metabolism. 97 (3): E367–E375. doi:10.1210/jc.2011-1997. PMID 22170725. S2CID 3162065.
- 1 2 3 Miller WL, Auchus RJ (April 2019). "The "backdoor pathway" of androgen synthesis in human male sexual development". PLOS Biology. 17 (4): e3000198. doi:10.1371/journal.pbio.3000198. PMC 6464227. PMID 30943210.
- 1 2 Shaw G, Renfree MB, Leihy MW, Shackleton CH, Roitman E, Wilson JD (October 2000). "Prostate formation in a marsupial is mediated by the testicular androgen 5 alpha-androstane-3 alpha,17 beta-diol". Proceedings of the National Academy of Sciences of the United States of America. 97 (22): 12256–12259. Bibcode:2000PNAS...9712256S. doi:10.1073/pnas.220412297. PMC 17328. PMID 11035809.
- 1 2 3 Mahendroo M, Wilson JD, Richardson JA, Auchus RJ (July 2004). "Steroid 5alpha-reductase 1 promotes 5alpha-androstane-3alpha,17beta-diol synthesis in immature mouse testes by two pathways". Molecular and Cellular Endocrinology. 222 (1–2): 113–120. doi:10.1016/j.mce.2004.04.009. PMID 15249131. S2CID 54297812.
- 1 2 3 4 5 Lee HG, Kim CJ (June 2022). "Classic and backdoor pathways of androgen biosynthesis in human sexual development". Annals of Pediatric Endocrinology & Metabolism. 27 (2): 83–89. doi:10.6065/apem.2244124.062. PMC 9260366. PMID 35793998. S2CID 250155674.
- ↑ Sharpe RM (August 2020). "Androgens and the masculinization programming window: human-rodent differences". Biochemical Society Transactions. 48 (4): 1725–1735. doi:10.1042/BST20200200. PMC 7458408. PMID 32779695.
- 1 2 3 4 O'Shaughnessy PJ, Antignac JP, Le Bizec B, Morvan ML, Svechnikov K, Söder O, et al. (February 2019). Rawlins E (ed.). "Alternative (backdoor) androgen production and masculinization in the human fetus". PLOS Biology. 17 (2): e3000002. doi:10.1371/journal.pbio.3000002. PMC 6375548. PMID 30763313.
- ↑ Josso N (May 2004). "The undervirilized male child: endocrine aspects". BJU Int. 93 (Suppl 3): 3–5. doi:10.1111/j.1464-410X.2004.04702.x. PMID 15086435. S2CID 35565574.
- 1 2 3 4 5 6 Reisch N, Taylor AE, Nogueira EF, Asby DJ, Dhir V, Berry A, et al. (October 2019). "Alternative pathway androgen biosynthesis and human fetal female virilization". Proceedings of the National Academy of Sciences of the United States of America. 116 (44): 22294–22299. Bibcode:2019PNAS..11622294R. doi:10.1073/pnas.1906623116. PMC 6825302. PMID 31611378.
- ↑ Sumińska M, Bogusz-Górna K, Wegner D, Fichna M (June 2020). "Non-Classic Disorder of Adrenal Steroidogenesis and Clinical Dilemmas in 21-Hydroxylase Deficiency Combined with Backdoor Androgen Pathway. Mini-Review and Case Report". International Journal of Molecular Sciences. 21 (13): 4622. doi:10.3390/ijms21134622. PMC 7369945. PMID 32610579.
- ↑ Biswas MG, Russell DW (June 1997). "Expression cloning and characterization of oxidative 17beta- and 3alpha-hydroxysteroid dehydrogenases from rat and human prostate". The Journal of Biological Chemistry. 272 (25): 15959–15966. doi:10.1074/jbc.272.25.15959. PMID 9188497.
- ↑ Muthusamy S, Andersson S, Kim HJ, Butler R, Waage L, Bergerheim U, Gustafsson JÅ (December 2011). "Estrogen receptor β and 17β-hydroxysteroid dehydrogenase type 6, a growth regulatory pathway that is lost in prostate cancer". Proceedings of the National Academy of Sciences of the United States of America. 108 (50): 20090–20094. Bibcode:2011PNAS..10820090M. doi:10.1073/pnas.1117772108. PMC 3250130. PMID 22114194.
- 1 2 Storbeck KH, Mostaghel EA (2019). "Canonical and Noncanonical Androgen Metabolism and Activity". Prostate Cancer. Advances in Experimental Medicine and Biology. Vol. 1210. Springer. pp. 239–277. doi:10.1007/978-3-030-32656-2_11. ISBN 978-3-030-32655-5. PMID 31900912. S2CID 209748543.
- ↑ Rizner TL, Lin HK, Penning TM (February 2003). "Role of human type 3 3alpha-hydroxysteroid dehydrogenase (AKR1C2) in androgen metabolism of prostate cancer cells". Chemico-Biological Interactions. 143–144: 401–409. doi:10.1016/s0009-2797(02)00179-5. PMID 12604227.
- ↑ Snaterse G, Mies R, van Weerden WM, French PJ, Jonker JW, Houtsmuller AB, et al. (June 2023). "Androgen receptor mutations modulate activation by 11-oxygenated androgens and glucocorticoids". Prostate Cancer and Prostatic Diseases. 26 (2): 293–301. doi:10.1038/s41391-022-00491-z. PMID 35046557. S2CID 246040148.
- ↑ Handelsman DJ, Cooper ER, Heather AK (April 2022). "Bioactivity of 11 keto and hydroxy androgens in yeast and mammalian host cells". The Journal of Steroid Biochemistry and Molecular Biology. 218: 106049. doi:10.1016/j.jsbmb.2021.106049. PMID 34990809. S2CID 245635429.
- ↑ Rege J, Nakamura Y, Satoh F, Morimoto R, Kennedy MR, Layman LC, et al. (March 2013). "Liquid chromatography-tandem mass spectrometry analysis of human adrenal vein 19-carbon steroids before and after ACTH stimulation". The Journal of Clinical Endocrinology and Metabolism. 98 (3): 1182–1188. doi:10.1210/jc.2012-2912. PMC 3590473. PMID 23386646.
- ↑ Campana C, Rege J, Turcu AF, Pezzi V, Gomez-Sanchez CE, Robins DM, Rainey WE (February 2016). "Development of a novel cell based androgen screening model". The Journal of Steroid Biochemistry and Molecular Biology. 156: 17–22. doi:10.1016/j.jsbmb.2015.11.005. PMC 4748855. PMID 26581480.
- ↑ Turcu AF, Nanba AT, Auchus RJ (2018). "The Rise, Fall, and Resurrection of 11-Oxygenated Androgens in Human Physiology and Disease". Hormone Research in Paediatrics. 89 (5): 284–291. doi:10.1159/000486036. PMC 6031471. PMID 29742491.
- ↑ Turcu AF, Auchus RJ (June 2015). "Adrenal steroidogenesis and congenital adrenal hyperplasia". Endocrinology and Metabolism Clinics of North America. Elsevier BV. 44 (2): 275–296. doi:10.1016/j.ecl.2015.02.002. PMC 4506691. PMID 26038201.
- ↑ Bacila IA, Elder C, Krone N (December 2019). "Update on adrenal steroid hormone biosynthesis and clinical implications" (PDF). Arch Dis Child. 104 (12): 1223–1228. doi:10.1136/archdischild-2017-313873. PMID 31175123. S2CID 182950024.
- ↑ Turcu AF, Rege J, Chomic R, Liu J, Nishimoto HK, Else T, et al. (June 2015). "Profiles of 21-Carbon Steroids in 21-hydroxylase Deficiency". The Journal of Clinical Endocrinology and Metabolism. 100 (6): 2283–2290. doi:10.1210/jc.2015-1023. PMC 4454804. PMID 25850025.
- ↑ Nguyen LS, Rouas-Freiss N, Funck-Brentano C, Leban M, Carosella ED, Touraine P, et al. (November 2019). "Influence of hormones on the immunotolerogenic molecule HLA-G: a cross-sectional study in patients with congenital adrenal hyperplasia". European Journal of Endocrinology. 181 (5): 481–488. doi:10.1530/EJE-19-0379. PMID 31505456. S2CID 202555340.
- ↑ Kawarai Y, Ishikawa H, Segawa T, Teramoto S, Tanaka T, Shozu M (May 2017). "High serum progesterone associated with infertility in a woman with nonclassic congenital adrenal hyperplasia". The Journal of Obstetrics and Gynaecology Research. 43 (5): 946–950. doi:10.1111/jog.13288. PMID 28188961. S2CID 22366495.
- 1 2 van Rooyen D, Yadav R, Scott EE, Swart AC (May 2020). "CYP17A1 exhibits 17αhydroxylase/17,20-lyase activity towards 11β-hydroxyprogesterone and 11-ketoprogesterone metabolites in the C11-oxy backdoor pathway". The Journal of Steroid Biochemistry and Molecular Biology. 199: 105614. doi:10.1016/j.jsbmb.2020.105614. PMID 32007561. S2CID 210955834.
- ↑ Schardein JL (1980). "Congenital abnormalities and hormones during pregnancy: a clinical review". Teratology. 22 (3): 251–70. doi:10.1002/tera.1420220302. PMID 7015547.
- ↑ Fiet J, Gueux B, Raux-DeMay MC, Kuttenn F, Vexiau P, Brerault JL, et al. (March 1989). "Increased plasma 21-deoxycorticosterone (21-DB) levels in late-onset adrenal 21-hydroxylase deficiency suggest a mild defect of the mineralocorticoid pathway". The Journal of Clinical Endocrinology and Metabolism. 68 (3): 542–547. doi:10.1210/jcem-68-3-542. PMID 2537337.
- ↑ Fiet J, Gueux B, Raux-Demay MC, Kuttenn F, Vexiau P, Gourmelen M, et al. (December 1989). "[21-deoxycortisol. A new marker of virilizing adrenal hyperplasia caused by 21-hydroxylase deficiency]". Presse Médicale. 18 (40): 1965–1969. PMID 2531882.
- ↑ Fiet J, Le Bouc Y, Guéchot J, Hélin N, Maubert MA, Farabos D, Lamazière A (March 2017). "A Liquid Chromatography/Tandem Mass Spectometry Profile of 16 Serum Steroids, Including 21-Deoxycortisol and 21-Deoxycorticosterone, for Management of Congenital Adrenal Hyperplasia". Journal of the Endocrine Society. 1 (3): 186–201. doi:10.1210/js.2016-1048. PMC 5686660. PMID 29264476.
- ↑ Greaves RF, Kumar M, Mawad N, Francescon A, Le C, O'Connell M, Chi J, Pitt J (October 2023). "Best Practice for Identification of Classical 21-Hydroxylase Deficiency Should Include 21 Deoxycortisol Analysis with Appropriate Isomeric Steroid Separation". Int J Neonatal Screen. 9 (4): 58. doi:10.3390/ijns9040058. PMC 10594498. PMID 37873849.
- ↑ Turcu AF, Nanba AT, Chomic R, Upadhyay SK, Giordano TJ, Shields JJ, et al. (May 2016). "Adrenal-derived 11-oxygenated 19-carbon steroids are the dominant androgens in classic 21-hydroxylase deficiency". European Journal of Endocrinology. 174 (5): 601–609. doi:10.1530/EJE-15-1181. PMC 4874183. PMID 26865584.
- ↑ Auchus RJ, Buschur EO, Chang AY, Hammer GD, Ramm C, Madrigal D, et al. (August 2014). "Abiraterone acetate to lower androgens in women with classic 21-hydroxylase deficiency". The Journal of Clinical Endocrinology and Metabolism. 99 (8): 2763–2770. doi:10.1210/jc.2014-1258. PMC 4121028. PMID 24780050.
- ↑ Turcu AF, Mallappa A, Nella AA, Chen X, Zhao L, Nanba AT, et al. (2021). "24-Hour Profiles of 11-Oxygenated C
19 Steroids and Δ5-Steroid Sulfates during Oral and Continuous Subcutaneous Glucocorticoids in 21-Hydroxylase Deficiency". Frontiers in Endocrinology. 12: 751191. doi:10.3389/fendo.2021.751191. PMC 8636728. PMID 34867794. - 1 2 Turcu AF, Mallappa A, Elman MS, Avila NA, Marko J, Rao H, et al. (August 2017). "11-Oxygenated Androgens Are Biomarkers of Adrenal Volume and Testicular Adrenal Rest Tumors in 21-Hydroxylase Deficiency". The Journal of Clinical Endocrinology and Metabolism. 102 (8): 2701–2710. doi:10.1210/jc.2016-3989. PMC 5546849. PMID 28472487.
- ↑ Schröder MA, Turcu AF, O'Day P, van Herwaarden AE, Span PN, Auchus RJ, et al. (January 2022). "Production of 11-Oxygenated Androgens by Testicular Adrenal Rest Tumors". The Journal of Clinical Endocrinology and Metabolism. 107 (1): e272–e280. doi:10.1210/clinem/dgab598. PMC 8684463. PMID 34390337.
- 1 2 Flück CE, Pandey AV (May 2014). "Steroidogenesis of the testis – new genes and pathways". Annales d'Endocrinologie. 75 (2): 40–47. doi:10.1016/j.ando.2014.03.002. PMID 24793988.
- ↑ Zachmann M (February 1996). "Prismatic cases: 17,20-desmolase (17,20-lyase) deficiency". The Journal of Clinical Endocrinology and Metabolism. 81 (2): 457–459. doi:10.1210/jcem.81.2.8636249. PMID 8636249.
- ↑ Batista RL, Mendonca BB (2022). "The Molecular Basis of 5α-Reductase Type 2 Deficiency". Sex Dev. 16 (2–3): 171–183. doi:10.1159/000525119. PMID 35793650. S2CID 250337336.
- ↑ Sultan C, Lumbroso S, Paris F, Jeandel C, Terouanne B, Belon C, Audran F, Poujol N, Georget V, Gobinet J, Jalaguier S, Auzou G, Nicolas JC (August 2002). "Disorders of androgen action". Semin Reprod Med. 20 (3): 217–28. doi:10.1055/s-2002-35386. PMID 12428202. S2CID 41205149.
- 1 2 Boettcher C, Flück CE (January 2022). "Rare forms of genetic steroidogenic defects affecting the gonads and adrenals". Best Practice & Research. Clinical Endocrinology & Metabolism. 36 (1): 101593. doi:10.1016/j.beem.2021.101593. PMID 34711511. S2CID 242536877.
- 1 2 du Toit T, Swart AC (September 2021). "Turning the spotlight on the C11-oxy androgens in human fetal development". The Journal of Steroid Biochemistry and Molecular Biology. 212: 105946. doi:10.1016/j.jsbmb.2021.105946. PMID 34171490. S2CID 235603586.
- ↑ Finkielstain GP, Vieites A, Bergadá I, Rey RA (2021). "Disorders of Sex Development of Adrenal Origin". Frontiers in Endocrinology. 12: 770782. doi:10.3389/fendo.2021.770782. PMC 8720965. PMID 34987475.
- ↑ de Hora M, Heather N, Webster D, Albert B, Hofman P (2023). "The use of liquid chromatography-tandem mass spectrometry in newborn screening for congenital adrenal hyperplasia: improvements and future perspectives". Frontiers in Endocrinology. 14: 1226284. doi:10.3389/fendo.2023.1226284. PMC 10578435. PMID 37850096.
- 1 2 3 4 5 6 du Toit T, Swart AC (2018). "Inefficient UGT-conjugation of adrenal 11β-hydroxyandrostenedione metabolites highlights C11-oxy C19 steroids as the predominant androgens in prostate cancer". Mol Cell Endocrinol. 461: 265–276. doi:10.1016/j.mce.2017.09.026. PMID 28939401. S2CID 6335125.
- ↑ Ventura-Bahena A, Hernández-Pérez JG, Torres-Sánchez L, Sierra-Santoyo A, Escobar-Wilches DC, Escamilla-Núñez C, Gómez R, Rodríguez-Covarrubias F, López-González ML, Figueroa M (2021). "Urinary androgens excretion patterns and prostate cancer in Mexican men". Endocr Relat Cancer. 28 (12): 745–756. doi:10.1530/ERC-21-0160. PMID 34520388. S2CID 237515025.
- 1 2 Luu-The V, Bélanger A, Labrie F (2008). "Androgen biosynthetic pathways in the human prostate". Best Practice & Research. Clinical Endocrinology & Metabolism. Elsevier BV. 22 (2): 207–221. doi:10.1016/j.beem.2008.01.008. ISSN 1521-690X. PMID 18471780.
- ↑ Storbeck KH, Bloem LM, Africander D, Schloms L, Swart P, Swart AC (2013). "11β-Hydroxydihydrotestosterone and 11-ketodihydrotestosterone, novel C19 steroids with androgenic activity: a putative role in castration resistant prostate cancer?". Mol Cell Endocrinol. 377 (1–2): 135–46. doi:10.1016/j.mce.2013.07.006. PMID 23856005. S2CID 11740484.
- ↑ Dimitrakov J, Joffe HV, Soldin SJ, Bolus R, Buffington CA, Nickel JC (2008). "Adrenocortical hormone abnormalities in men with chronic prostatitis/chronic pelvic pain syndrome". Urology. 71 (2): 261–6. doi:10.1016/j.urology.2007.09.025. PMC 2390769. PMID 18308097.
- ↑ du Toit T, Swart AC (2020). "The 11β-hydroxyandrostenedione pathway and C11-oxy C21 backdoor pathway are active in benign prostatic hyperplasia yielding 11keto-testosterone and 11keto-progesterone". The Journal of Steroid Biochemistry and Molecular Biology. 196: 105497. doi:10.1016/j.jsbmb.2019.105497. PMID 31626910. S2CID 204734045.
- ↑ Masiutin MG, Yadav MK (2022). "Letter to the editor regarding the article "Adrenocortical hormone abnormalities in men with chronic prostatitis/chronic pelvic pain syndrome"". Urology. 169: 273. doi:10.1016/j.urology.2022.07.051. ISSN 0090-4295. PMID 35987379. S2CID 251657694.
- ↑ Dimitrakoff J, Nickel JC (2022). "Author reply". Urology. 169: 273–274. doi:10.1016/j.urology.2022.07.049. ISSN 0090-4295. PMID 35985522. S2CID 251658492.
- ↑ Eckstein B, Borut A, Cohen S (April 1987). "Metabolic pathways for androstanediol formation in immature rat testis microsomes". Biochimica et Biophysica Acta (BBA) – General Subjects. 924 (1): 1–6. doi:10.1016/0304-4165(87)90063-8. PMID 3828389.
- ↑ Penning TM (June 1997). "Molecular endocrinology of hydroxysteroid dehydrogenases". Endocrine Reviews. 18 (3): 281–305. doi:10.1210/edrv.18.3.0302. PMID 9183566. S2CID 29607473.
- ↑ van Rooyen D, Gent R, Barnard L, Swart AC (April 2018). "The in vitro metabolism of 11β-hydroxyprogesterone and 11-ketoprogesterone to 11-ketodihydrotestosterone in the backdoor pathway". The Journal of Steroid Biochemistry and Molecular Biology. 178: 203–212. doi:10.1016/j.jsbmb.2017.12.014. PMID 29277707. S2CID 3700135.
This article incorporates text available under the CC BY-SA 3.0 license.