Llullaillaco | |
---|---|
![]() Llullaillaco from the east | |
Highest point | |
Elevation | 6,739 m (22,110 ft) ![]() |
Prominence | 2,344 m (7,690 ft) ![]() |
Isolation | 264.53 km (164.37 mi) ![]() |
Listing | Ultra |
Coordinates | 24°43′00″S 68°32′00″W / 24.71667°S 68.53333°W[1] |
Geography | |
![]() ![]() Llullaillaco Location in Chile (on border with Argentina) | |
Location | Chile and Argentina |
Parent range | Andes, Puna de Atacama |
Geology | |
Age of rock | Pleistocene |
Mountain type | Stratovolcano |
Last eruption | May 1877[2] |
Climbing | |
First ascent | 1950, but previous climbs by Inca[3] |
Llullaillaco (Spanish pronunciation: [ʎuʎajˈʎako]) is a dormant stratovolcano at the border of Argentina (Salta Province) and Chile (Antofagasta Region). It lies in the Puna de Atacama, a region of tall volcanic peaks on a high plateau close to the Atacama Desert, one of the driest places in the world. It is the second highest active volcano in the world after Ojos del Salado.
Llullaillaco formed during two different phases in the Pleistocene-Holocene out of dacitic lava flows. The oldest rocks are about 1.5 million years old. About 150,000 years ago, the volcano's southeastern flank collapsed, generating a debris avalanche that reached as far as 25 kilometres (16 mi) from the summit. The youngest dated rocks were erupted 5,600 ± 250 years ago in the summit region, but there are reports of activity from the 19th century.
The mountain's first recorded climb was in 1950, but traces of earlier climbs and a number of archaeological sites were found on the mountain and at its feet; Llullaillaco marks the highest archaeological site in the world. In 1999, the mummified remains of three children, known as the Children of Llullaillaco, were found at its summit. They are presumed to have been human sacrifices. Mice have been observed in the summit region; aside from humans, they are the highest finding of a mammal in the world.
Name
The name Llullaillaco is derived from the Quechua word llulla meaning "false", "lie" or "deceitful" and yaku or llaco meaning "water".[4] This name probably refers to the meltwater from snow, which flows down the slopes but then is absorbed into the soil;[5] normally mountains are sources for water.[4] It is possible that it instead refers to the precipitation regime, which starting from Llullaillaco becomes dominated by winter precipitation.[6] Another translation in Aymara is "hot water".[7]
Geography and geology
Volcanism in the Andes is caused by the subduction of the Nazca Plate and the Antarctic Plate beneath the South America Plate. The Nazca Plate subducts at a speed of 7–9 centimetres per year (2.8–3.5 in/year) and the Antarctic Plate at a speed of 2 centimetres per year (0.79 in/year). Volcanism does not occur along a continuous chain; there are four separate regions named: the Northern Volcanic Zone, the Central Volcanic Zone, the Southern Volcanic Zone, and the Austral Volcanic Zone. The formation of magma results from the release of water and other volatile material from the subducting plate, which is then injected into the above-lying mantle wedge. The volcanic zones are separated by areas where the subducting plate subducts at a flatter angle and volcanism is absent. The Peruvian flat slab between the Northern and the Central Volcanic Zones is associated with the subduction of the Nazca Ridge, the Pampean flat slab between the Central and the Southern Volcanic Zone is associated with the subduction of the Juan Fernandez Ridge, and the Patagonian volcanic gap between the Southern and the Austral Volcanic Zone is associated with the Chile Triple Junction.[8]
About 178 volcanoes are found in the Andes, 60 of which have been active in historical times. In addition, large calderas and monogenetic volcanoes exist in the Andes.[8]
Local setting
Llullaillaco is part of the Central Volcanic Zone.[9] At least 44 volcanic centres with historical activity and 18 large caldera-forming volcanoes have been identified in the Central Volcanic Zone.[8] Volcanism in the Central Volcanic Zone mostly occurs on the Altiplano and the Cordillera Occidental. A number of volcanoes there reach heights of over 6,000 metres (20,000 ft) above sea level. Large Miocene ignimbrites that cover large surfaces are also part of the regional geology.[10] Llullaillaco is located about 300 kilometres (190 mi) east of the Peru-Chile Trench.[11] The Wadati-Benioff zone lies at 180 kilometres (110 mi) depth.[12]
Llullaillaco is located in the northwestern Argentine Andes,[13] towards the southern end of the Puna.[14] It lies in the Puna de Atacama, a region of very high volcanic peaks on a high plateau[15] close to the Atacama Desert,[16] one of the driest places in the world.[17] The border between Argentina (Salta Province) and Chile crosses the volcano.[2]
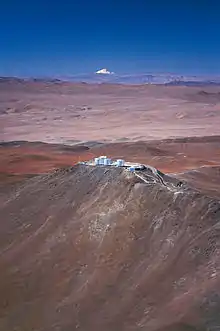
The volcano is an imposing mountain, rising by 3,800 metres (12,500 ft) above the Salar de Punta Negra 36 kilometres (22 mi) away.[18] With a summit height of 6,723 metres (22,057 ft),[19][1] or alternatively 6,739 metres (22,110 ft)[2] or 6,752 metres (22,152 ft),[20] it is the second highest active volcano in the world after Ojos del Salado (6,887 metres (22,595 ft), 27°07′S 68°33′W / 27.117°S 68.550°W).[19][1] The height of the mountain and the clear air in the region make Llullaillaco visible from Cerro Paranal, 189 kilometres (117 mi) away as measured through Google Maps.[21] The Nevados de Cachi mountains and Ojos del Salado 250 kilometres (160 mi) farther south are visible from Lllullaillaco.[22] The region is dry and located at high altitudes, making work in the area difficult.[18]
17 kilometres (11 mi) farther east[2] of Llullaillaco lies the Miocene Cerro Rosado volcano (5,383 metres (17,661 ft)). It erupted dacitic lava flows on its northeastern and southern flanks during the Pliocene-Quaternary.[23] 20 kilometres (12 mi) east of Llullaillaco,[18] and behind Cerro Rosado is the Salar de Llullaillaco (3,750 metres (12,300 ft) [24]), a salt pan with warm springs at its western and southwestern shores. There are three abandoned borate mines Mina Amalia and the salt mines Mina Luisa and Mina Maria[23] and the recent lithium prospect "Proyecto Mariana" at Salar de Llullaillaco.[25] Mitral mountain (5,015 metres (16,453 ft)) lies southwest of Llullaillaco and is of Miocene age. It features an eroded crater that opens to the northwest.[26][27] Iris mountain (5,461 metres (17,917 ft)) north of Llullaillaco is constructed of Pliocene rocks.[26] Other volcanoes in the neighbourhood are Dos Naciones, Cerro Silla, and Cerro 5074.[28] Llullaillaco is associated with a local crustal upwarp.[29]
The volcano
Llullaillaco is a stratovolcano which rises 2,200 metres (7,200 ft) above the surrounding terrain.[1][19] The edifice has an elliptical shape with dimensions of 23 by 17 kilometres (14 mi × 11 mi) and has a volume of about 50 cubic kilometres (12 cu mi)[19][30]-37 cubic kilometres (8.9 cu mi).[31] It is formed by thick dacitic lava flows erupted during the Quaternary.[32] These flows extend away from the volcano and form its summit. An older unit is formed by ignimbrites and pyroclastic flows.[12] Older lava flows extend west from the volcano and are partly buried by sediments closer to the edifice. These flows reach lengths of 20 kilometres (12 mi) and form about 70% of the surface of the volcano.[33]
The slopes of the volcano are fairly steep, with an altitude drop of 1,800 metres (5,900 ft) over only 3 kilometres (1.9 mi) horizontal distance.[18][34] The slopes high up are steeper than those at lower altitudes.[35] A crater at 6,100 metres (20,000 ft) altitude was formed early in the development of the Llullaillaco volcano.[36] Erosion has reduced it to a plateau.[33] This crater is filled with snow and ice. The ice shows evidence of recent geothermal heating.[37]
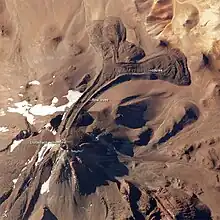
The summit of Llullaillaco is formed by a small cone with about four associated lava domes,[33] which reach lengths of 1–3 kilometres (0.62–1.86 mi) and have abrupt walls.[38] Large dacitic coulees emanate from the summit of the volcano and are young in appearance.[39] One extends north of the volcano and the other one south.[2] Their overall length is 4.5–8 kilometres (2.8–5.0 mi) and their flow fronts are up to 15 metres (49 ft) thick. Morphologically, these flows are reddish-black aa lava flows and feature blocks with sizes of 5 metres (16 ft).[33] The southern lava flow is 6 kilometres (3.7 mi) long and was fairly viscous when it was erupted.[39] It almost reaches a road southwest of the volcano.[26] These flows feature levees and ridges. At first they were considered to be Holocene, but argon-argon dating indicates they are of late Pleistocene age.[2] Some traces of glacier activity are found in the summit area.[36]
At least two stages of construction are recognized, Llullaillaco I and Llullaillaco II. The first stage originated from two centres and is now extensively degraded by glaciation and hydrothermal alteration.[40] This applies especially to the 5,561 metres (18,245 ft) high Azufrera Esperanto mountain 5 kilometres (3.1 mi) north of Llullaillaco, where little original volcanic substance is preserved and where erosion has exposed deeply altered white rock.[33] Llullaillaco II on the southern and northeastern flank is better preserved; the toes of the lava flows reach thicknesses of 500 metres (1,600 ft).[39] Its lava flows are less extensive than the ones of Llullaillaco I.[12] Pyroclastic flow deposits with a composition similar to Llullaillaco II are found on the southern slope of the volcano and may have formed before the lava eruptions began.[38]
Volcanism in the Central Volcanic Zone may be affected by deep-seated lineaments, which control where volcanoes and geothermal systems form.[41] Such lineaments extend diagonally across the volcanic arc and are accompanied by volcanic manifestations at substantial distances from the arc.[42] One of these lineaments, the Archibarca, runs beneath Llullaillaco and is also associated with the Escondida copper deposit, [43] Corrida de Cori,[41] Archibarca, Antofalla,[44] and the Galán caldera.[43] Other lineaments include the Calama-El Toro.[42]
Several dry valleys originate on Llullaillaco, including Quebrada de las Zorritas on the north-northeastern slope, Quebrada El Salado and Quebrada Llullaillaco on the northwestern slope, and Quebrada La Barda on the southwestern slope.[45] The Quebrada Llullaillaco drains into the Salar de Punta Negra northwest of the volcano.[46] The volcano is relatively unaffected by water erosion,[35] water is only episodically present on the mountain.[47] On the northwestern flank there is a 0.9 hectares (2.2 acres) lake called the "Lago Llullaillaco" on the mountain; with an elevation of 6,170 metres (20,240 ft)[48] it is one of the highest lakes in the world.[49][50]
Two abandoned sulfur mines can be found north and south of Llullaillaco.[23] The northern mine is known as Azufrera Esperanto and associated with an area of hydrothermal alteration. A path or road leads up to that mine from northwest.[27]
- Llullaillaco during sunset
- Llullailaco with a road leading to the mountain.
- Llullaillaco from the base camp.
- Lava flow from Llullaillaco
- Llullaillaco in Salta
Debris avalanche
A major landslide occurred in the volcano's history during the late Pleistocene, probably triggered by volcanic activity.[32] This landslide descended the eastern-southeastern flanks of the volcano into Argentina,[39][2] first over a steep slope of 20° on the volcano,[51] split around Cerro Rosado and entered the Salina de Llullaillaco 25 kilometres (16 mi) east of the summit,[39] extending up to 5 kilometres (3.1 mi) into the salar.[52] The toe of the avalanche reaches a thickness of 10 metres (33 ft) above the salar in the avalanche's southern lobe. Part of the avalanche was channelled in a valley between Cerro Rosado and an unnamed volcano farther south.[53] When it reached Cerro Rosado, the avalanche climbed about 400 metres (1,300 ft) and mostly continued to flow southeast into the main avalanche path, with only a small flow continuing northeastwards. Part of the run-up avalanche later collapsed backwards over the main avalanche deposit.[54] Unlike Socompa farther north, a landslide scar is only poorly developed at Llullaillaco despite the large size of the collapse;[55] it was largely filled in later by lava flows and volcaniclastic debris.[56]
This landslide has been subdivided into four facies and features landforms like levees up to 50 metres (160 ft) high,[57] longitudinal ridges and a run-up mark on Cerro Rosado.[52] Such ridges may be associated with uneven underlying terrain.[58] The surface of the slide is covered by lava bombs less than 1 metre (3 ft 3 in) long, blocks exceeding 2 metres (6 ft 7 in) width, cobbles, and gravel-like rocks.[59] Close to the collapse scarp on Llullaillaco the largest blocks with sizes of up to 20 metres (66 ft) are found.[54] Overall, the margins of the landslide are very crisp and the surface covered by hummocks.[60]
The landslide deposit covers a surface of about 165 square kilometres (64 sq mi).[39] Its volume has been estimated at 1–2 cubic kilometres (0.24–0.48 cu mi) and the speed at 45–90 metres per second (150–300 ft/s). This speed range is comparable to that of the Colima, Lastarria, and Mount St. Helens debris avalanches.[61] The landslide occurred no later than 156,000 – 148,000 ± 5,000 years ago,[39] it might coincide with the 48,000 year old lava flow.[51] Some volcanic rocks were still hot at the time of the collapse, indicating that volcanic activity occurred immediately before the collapse.[55] A smaller undated avalanche occurred on the northeastern flank.[39]
Such landslides are common at volcanoes, where they are favoured by the steep edifices that form from lava flows being stacked on top of each other. It is not usually known what triggers the collapse, although eruption-associated earthquakes are suspected to play a role. Other volcanoes in the region with sector collapses are: Lastarria, Ollague, San Pedro, Socompa, and Tata Sabaya.[62] The Mellado and Cerro Rosado volcanoes close to Llullaillaco also display evidence of sector collapses.[56]
Glaciers and periglacial phenomena
.jpg.webp)
In 2006 the General Water Directorate of Chile stated that there were seven separate ice bodies on the mountain.[63] Small glaciers are found above 6,000 metres (20,000 ft) altitude.[32] Other sources contend there are no glaciers on Llullaillaco, which would make it the highest mountain in the world without one;[64] although the existence of an ice slab on the western slope was reported in 1958[65] between 5,600–6,500 metres (18,400–21,300 ft) elevation[66] and in 1992 there was an ice body which however lacked all evidence of glacial motion.[67] However, Llullaillaco has snow fields[34] tied to permafrost in the ground.[68] 1–1.5 metres (3 ft 3 in – 4 ft 11 in) high penitentes occur above 5,000 metres (16,404 ft) altitude,[69] especially in more sheltered areas.[70] 1 metre (3 ft 3 in) high penitentes were observed in 1954.[71] Snow covers the terrain above 5,400–5,800 metres (17,700–19,000 ft) altitude.[3] Gullies that are found in the area probably result from meltwater runoff.[28] The snowfields on Llullaillaco supply water to the Salar de Punta Negra.[72] The lack of glaciers is a consequence of the dry climate,[73] as the high insolation and dry air allow all snow to evaporate before it can form glaciers.[74]
The existence of a cirque on the northwestern slope has been postulated.[75] It was once believed that Llullaillaco had experienced three large glaciations,[76] but the "moraines" are actually mudflow deposits.[77] However, during the Pleistocene glaciers reached down to 5,100 metres (16,700 ft) altitude on the northwestern slope. Lava flows have overrun some of the glaciated surfaces and it is possible that lava flows induced the melting of glaciers.[77] A number of glacial landforms elsewhere were destroyed by mudflows during the time of Llullaillaco's last activity.[78] Llullaillaco may not have been glaciated at all during the Pleistocene.[79]
Periglacial phenomena are observed on Llullaillaco, commencing at 4,300 metres (14,100 ft) altitude and reaching their maximum around 5,100–5,400 metres (16,700–17,700 ft) on the Chilean and 5,350–5,700 metres (17,550–18,700 ft) on the Argentine side. There, solifluction and cryoplanation surfaces are developed,[80] including lobe-shaped ground and block ramparts.[81] These landforms have been mapped on the northwestern side of the volcano.[82] Patterned ground is also common.[83] Permafrost is found at higher altitudes.[80] Cryoplanation and solifluction landforms are also observed on Iris and Mitral.[84] Other than on periglacially influenced terrain, the ground at Llullaillaco is formed mostly by lavic rocks and block debris, which are frequently buried by tephra.[85]
Composition
Llullaillaco has mostly erupted dacites with medium potassium content, with rocks becoming more felsic the younger they are.[86] Rock samples taken from Llullaillaco are mostly porphyritic or vitrophyric, with a glassy or microcrystalline matrix. Phenocrysts are mostly plagioclase, with mafic phenocrysts being dominated by orthopyroxene and smaller amounts of biotite, clinopyroxene, and hornblende. Ilmenite, magnetite, and sulfide minerals are also present, magnetite especially in the more oxidized older lavas.[39] Some phenocrysts show evidence of a complex history.[87] Quartz is rare. Older lavas have developed a red colour and contain oxidized iron in form of hematite.[88] Lavas from Llullaillaco I are grey.[12] The content of SiO
2 is 65–67%.[30]
The rocks belong to the calc-alkaline series.[12] Trace element data are typical for Central Volcanic Zone rocks.[86] High potassium content is typical for shoshonite-like lavas erupted at large distance from the trenches.[11]
The composition may reflect magma differentiation in a solitary magma chamber, but with occasional replenishment with more primitive magma.[86] Magma mixing and plagioclase crystallization occurred in it.[89] A lithospheric structure probably directed magma flows over long time periods along the same pathway.[61] The depth of the magma source probably varied over the history of the volcano.[90] The total magma output at Llullaillaco is about 0.05 cubic kilometres per millennium (0.012 cu mi/ka)[30]-0.02–0.04 cubic kilometres per millennium (0.0048–0.0096 cu mi/ka).[31]
Geological history
The terrain around Llullaillaco consists of andesite and dacite lavas and pyroclastics of Miocene to Pliocene age.[23] Some Oligocene-Miocene layers are exposed in the Quebrada de las Zorritas.[84] Dates obtained by argon-argon dating range between 11.94 ± 0.13 and 5.48 ± 0.07 million years ago. 15 kilometres (9.3 mi) west of Llullaillaco do Paleozoic granites and volcanite rocks crop out. Elsewhere these layers are buried by Cenozoic rocks.[40] There are several faults in the region, such as the Imilac-Salina del Fraile lineament whose movement has influenced the activity of Llullaillaco.[91]
Research by J.P. Richards and M. Villeneuve has allowed the determination of the geological history of the region. Had volcanism during the Eocene and Oligocene been centered on the Precordillera, increased subduction of the Nazca Plate during the late Oligocene caused the volcanic arc to broaden to about 250 kilometres (160 mi). 25 million years ago, the "Quechua event" triggered the uplift of the Puna-Altiplano, a highland covering a surface area of 500,000 square kilometres (190,000 sq mi) and reaching an altitude of 3,700 metres (12,100 ft). In the late Miocene-Pliocene a phase of strong ignimbrite volcanism occurred. About 2 million years ago, the "Diaguita deformation" was characterized by a change in the deformation regimen from crustal shortening to strike-slip faulting and of volcanism from voluminous felsic eruptions to isolated stratovolcanoes and back-arc mafic volcanism. A slowdown in the subduction may have caused this change. Nowadays most volcanism occurs at the western edge of the Puna, where volcanoes such as Lascar and Llullaillaco formed.[9][42]
Climate and biota
The climate at Llullaillaco is cold and dry.[3] The average temperature is about −13 °C (9 °F),[13] with temperature maximums ranging −8 – −13 °C (18–9 °F) between summer and winter.[92] Ground temperatures however can reach 12.5 °C (54.5 °F) during the day in summer.[93] The temperature of the ground fluctuates strongly during the day.[94]
Llullaillaco in 2004 became the site of the highest weather station in the world.[22] The climate is extremely sunny on account of the lack of a cloud cover, the high altitude, and the close coincidence between summer solstice with the day where Earth is closest to the Sun.[95] Snowfall can occur down to altitudes of 4,000 metres (13,123 ft).[80] Precipitation is episodic to the point that it is difficult to give average values.[96] It is most often associated with either convective or cyclonic activity during summer and winter respectively.[97]
Between 24 and 25° degrees southern latitude the Andean Dry Diagonal crosses the Andes. At this latitude, half of the precipitation falls in summer and the other half in winter.[18] The formation of this dry diagonal is an effect both of the rainshadow effect of the Andes, air subsidence within South Pacific High, and the cold Humboldt Current off the Pacific coast. This is the reason why the Atacama Desert exists.[96][98] The extremely arid climate at Llullaillaco is also a consequence of these climatic effects.[96]
Vegetation is scarce in the arid climate.[32] Vegetation at Llullaillaco begins at 3,700–3,800 metres (12,100–12,500 ft) altitude with Acantholippia punensis, Atriplex imbricata, and Cristaria andicola. They are joined at 3,900 metres (12,800 ft) altitude by Stipa frigida which can be found up to 4,910 metres (16,110 ft) of altitude. The maximum vegetation density is found around 4,250 metres (13,940 ft) with 12% of the surface and decreases thereafter, probably due to the low temperatures. At this altitude, Adesmia spinosissima, Fabiana bryoides, Mulinum crassifolium, and Parastrephia quadrangularis are found in addition to the previously mentioned plants.[99][100] Above 5,000 metres (16,000 ft) elevation there is no vegetation.[69] Mice were filmed at 6,205 metres (20,358 ft) in 2013, and an expedition in February 2020 found mice all the way to the summit, where a mouse of the species Phyllotis xanthopygus was captured. This is the highest altitude in the world at which a mammal has been found to live.[101]
Weakly developed[102] fungal[103] and bacterial communities have been found in the soils at Llullaillaco,[102] with better developed algal[104] and cyanobacterial communities found on the penitentes;[105] some soil microbes may depend on volcanic carbon monoxide.[102] Algae, lichens, microbial mats and vascular plants grow around and in Lago Llullaillaco.[106] The environmental conditions in these soils are among the most extreme on the planet,[107] with aridity, strong UV radiation, daily freeze-thaw cycles and a lack of nutrients. Organic material transported on the mountain by winds may be the principal source of food for some of these microorganisms.[108] Air pollution from the Escondida copper mine may reach Llullaillaco and threaten ecosystems there.[109]
Dry valleys and sheltered areas feature grasses.[32] A permanent spring is found in Quebrada de las Zorritas.[99] Deposits in this valley indicate that between 2,436.8 ± 49 and 1,593.1 ± 36 radiocarbon years ago runoff was more intense.[110]
- Llullaillaco from Salar Rio Grande
- Llullaillaco from Salar de Aguas Calientes IV
Archaeology
Llullaillaco was first climbed by Incas centuries ago.[111] In 1999,[112] a team of archeologists,[113] led by Johan Reinhard,[114] found three mummies of children on the top of Llullaillaco.[13] At the time of their death they were six, seven, and fifteen years old.[113] They were ostensibly human sacrifices offered to the gods of the Incan pantheon on mountaintops.[115] In 2003 they were at the Catholic University of Salta,[113] but starting from 2007 they can be found at the Museum of High Altitude Archaeology of Argentina.[116]
The mummies were found buried 1.7 metres (5 ft 7 in) beneath an 11 by 6 metres (36 ft × 20 ft) large platform.[13][3] The existence of ruins in the summit area had been reported during the first modern climb of the volcano in 1950.[3] Because of the findings, the summit area of the volcano in 2001 was classified as a Lugar Histórico Nacional by the government of Argentina.[117]
Other archeological objects found along with the mummies included: headdresses with feathers, pottery, statues made out of gold and silver and textiles.[13] A total of 145 objects were found together with the mummies.[118] The hairs found with the mummies and as offerings accompanying the mummies have been subject of research to establish their background.[119]
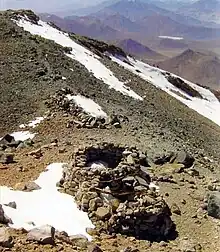
At least three paths lead up to Llullaillaco. Two of them meet at a tambo or inn at an altitude of 5,200 metres (17,100 ft), while the third passes through a cementerio (cemetery) where 16 bodies were found in 1972 before reaching the tambo. From the tambo another path leads up the mountain, past two further archeological sites to 6,000 metres (20,000 ft) of altitude.[3] This path is an Inca ceremonial path with an initial width of 2–1.5 metres (6 ft 7 in – 4 ft 11 in), narrowing in steeper reaches.[120] The path is marked by cairns, probably to indicate where the path is when the volcano is covered by snow. The path ends at 6,500 metres (21,300 ft) altitude at the Portezuelo del Inca ruins, where a staircase begins. From these ruins one path goes to a cemetery at 6,715 metres (22,031 ft) altitude and another to the summit platform. The other path reaches two subrectangular walls 20 metres (66 ft) farther up, and continues to the platform where the mummies were found.[3] These are the highest archeological sites in the world.[112] The paths were discovered by Mathias Rebitsch in 1958.[121]
Llullaillaco appears to have been the most important Inca sacred mountain in the region.[122] Archeological sites are also found in the valleys that drain Llullaillaco towards the Salar de Punta Negra,[123] including the Quebrada Llullaillaco and the Quebrada de las Zorritas.[118] The paths connect with the major north–south axis that runs between San Pedro de Atacama and Copiapo.[124] This axis is associated with the extensive Inca road system.[125]
Eruptive history
The volcano is about 1.45 ± 0.41 million years old,[31] and Llullaillaco I is of early Pleistocene age.[40] The oldest date, 1.5 ± 0.4 million years ago, was determined on a lava flow northwest of Llullaillaco's main edifice.[27] Two dates obtained on lava flows of Llullaillaco II are 401,000 ± 6,000 and 1,500,000 ± 400,000 years ago, based on argon-argon dating and potassium-argon dating respectively.[39] The northern young lava flow is less than one million years old based on potassium-argon dating, the southern flow is 48,000 ± 12,000 years old based on argon-argon dating on biotite.[39] Surface exposure dating based on helium has yielded ages of 41,000 ± 1,000 years for Llullaillaco I at over 5,000 metres (16,000 ft) altitude, of 5,600 ± 250 years for Llullaillaco II at an altitude of over 6,000 metres (20,000 ft)[77] and of 930 ± 140 years.[126]
Llullaillaco has been active in historical time,[127] with the last eruption occurring in the late 19th century.[88] Eruptions were recorded in February 1854, September 1868, and May 1877, involving two explosive eruptions and one with lava flows.[2] According to a report in 1899, during the 1868 eruption large fissures opened on its slopes.[5] The last eruption consisted of smoking reported at the time of the 1877 Iquique earthquake.[128] Other reports mention eruptions in 1920, 1931, 1936 and 1960.[129]
The volcano is currently considered dormant[19] and there are no known fumaroles.[130] Llullaillaco is considered Argentina's 16th most dangerous volcano out of 38.[131] Future eruptive activity may result in the emission of pyroclastic flows and lava flows[129] and may cause sector collapses, although they would be a small danger to human life, given that the area is sparsely inhabited.[51]
See also
References
- 1 2 3 4 Richards & Villeneuve 2001, p. 78.
- 1 2 3 4 5 6 7 8 "Llullaillaco". Global Volcanism Program. Smithsonian Institution.
- 1 2 3 4 5 6 7 Mignone, Pablo (2010). "Ritualidad estatal, capacocha y actores sociales locales: El Cementerio del volcán Llullaillaco". Estudios Atacameños (in Spanish) (40): 43–62. doi:10.4067/S0718-10432010000200004. hdl:11336/14124. ISSN 0718-1043.
- 1 2 Vitry 2016, p. 5.
- 1 2 Francisco Astaburuaga Cienfuegos (1899). Diccionario Geográfico de la República de Chile (in Spanish). Imp. de F. A. Brockhaus. p. 399.
- ↑ Sanhueza, Cecilia; Berenguer, José; González, Carlos; González, Cristián; Cortés, Juan; Martín, Sergio; Cruz, Jimena; Sanhueza, Cecilia; Berenguer, José; González, Carlos; González, Cristián; Cortés, Juan; Martín, Sergio; Cruz, Jimena (September 2020). "Saywas y Geografía Sagrada en el Qhapaq Ñan del Despoblado de Atacama". Chungará (Arica). 52 (3): 485–508. doi:10.4067/S0717-73562020005001801. ISSN 0717-7356.
- ↑ Bobylyova, E. S.; Сергеевна, Бобылева Елена (15 December 2016). "Structural-and-Semantic Analysis of Oronyms of Chile, Структурно-семантический анализ оронимов Чили". RUDN Journal of Language Studies, Semiotics and Semantics, Вестник Российского университета дружбы народов. Серия: Теория языка. Семиотика. Семантика (in Russian) (2): 126. ISSN 2411-1236.
- 1 2 3 Stern, Charles R. (December 2004). "Active Andean volcanism: its geologic and tectonic setting". Revista Geológica de Chile. 31 (2). doi:10.4067/S0716-02082004000200001.
- 1 2 Richards & Villeneuve 2001, p. 89.
- ↑ Gardeweg, Cornejo & Davidson 2010, p. 22.
- 1 2 Richards & Villeneuve 2001, p. 94.
- 1 2 3 4 5 Schröder & Schmidt 1997, p. 229.
- 1 2 3 4 5 Previgliano et al. 2003, p. 1473.
- ↑ Richards & Villeneuve 2002, p. 171.
- ↑ Zeil, Werner (December 1964). "Die Verbreitung des jungen Vulkanismus in der Hochkordillere Nordchiles". Geologische Rundschau (in German). 53 (2): 736. Bibcode:1964GeoRu..53..731Z. doi:10.1007/bf02054561. ISSN 0016-7835. S2CID 128979648.
- ↑ Costello, Elizabeth K.; Halloy, Stephan R. P.; Reed, Sasha C.; Sowell, Preston; Schmidt, Steven K. (1 February 2009). "Fumarole-Supported Islands of Biodiversity within a Hyperarid, High-Elevation Landscape on Socompa Volcano, Puna de Atacama, Andes". Applied and Environmental Microbiology. 75 (3): 735–747. Bibcode:2009ApEnM..75..735C. doi:10.1128/AEM.01469-08. ISSN 0099-2240. PMC 2632159. PMID 19074608.
- ↑ Rech, Jason A.; Currie, Brian S.; Michalski, Greg; Cowan, Angela M. (2006). "Neogene climate change and uplift in the Atacama Desert, Chile". Geology. 34 (9): 763. Bibcode:2006Geo....34..761R. doi:10.1130/G22444.1. ISSN 0091-7613.
- 1 2 3 4 5 Schröder & Schmidt 1997, p. 225.
- 1 2 3 4 5 Gardeweg, Cornejo & Davidson 2010, p. 24.
- ↑ Biggar, John (2020). The Andes - A Guide for Climbers and Skiers (5th ed.). Andes. p. 211. ISBN 978-0-9536087-6-8.
- ↑ Hanuschik, R. (2013-03-01). "Llullaillaco and Paranal's Skyline". The Messenger. 151: 58–61. Bibcode:2013Msngr.151...58H. ISSN 0722-6691.
- 1 2 Kostka 2006, p. 54.
- 1 2 3 4 Richards & Villeneuve 2001, pp. 80–81.
- ↑ Schröder & Schmidt 1997, p. 227.
- ↑ Romeo, Gustavo; Argento, Melisa; Gamba, Martina; Kazimierski, Martín; Puente, Florencia; Santos, Elaine; Slipak, Ariel; Urrutia, Santiago; Zicari, Julián (2019), Fornillo, Bruno (ed.), "Riesgo ambiental e incertidumbre en la producción del litio en salares de Argentina, Bolivia y Chile", Litio en Sudamérica, Geopolítica, energía y territorios, CLACSO, p. 236, ISBN 978-987-47280-0-5, JSTOR j.ctvtxw25t.10, retrieved 2020-12-07
- 1 2 3 Schröder & Schmidt 1997, p. 230.
- 1 2 3 Gardeweg, Cornejo & Davidson 2010, p. 25.
- 1 2 Richards & Villeneuve 2002, p. 175.
- ↑ Pritchard, M.E.; de Silva, S.L.; Michelfelder, G.; Zandt, G.; McNutt, S.R.; Gottsmann, J.; West, M.E.; Blundy, J.; Christensen, D.H.; Finnegan, N.J.; Minaya, E.; Sparks, R.S.J.; Sunagua, M.; Unsworth, M.J.; Alvizuri, C.; Comeau, M.J.; del Potro, R.; Díaz, D.; Diez, M.; Farrell, A.; Henderson, S.T.; Jay, J.A.; Lopez, T.; Legrand, D.; Naranjo, J.A.; McFarlin, H.; Muir, D.; Perkins, J.P.; Spica, Z.; Wilder, A.; Ward, K.M. (29 March 2018). "Synthesis: PLUTONS: Investigating the relationship between pluton growth and volcanism in the Central Andes". Geosphere. 14 (3): 971. Bibcode:2018Geosp..14..954P. doi:10.1130/GES01578.1. hdl:1983/cf804ce1-dcfa-4abf-b2e3-0f267f7feed1. ISSN 1553-040X.
- 1 2 3 Klemetti, Erik W.; Grunder, Anita L. (24 July 2007). "Volcanic evolution of Volcán Aucanquilcha: a long-lived dacite volcano in the Central Andes of northern Chile". Bulletin of Volcanology. 70 (5): 647. Bibcode:2008BVol...70..633K. doi:10.1007/s00445-007-0158-x. S2CID 140668081.
- 1 2 3 Grosse, Pablo; Orihashi, Yuji; Guzmán, Silvina R.; Sumino, Hirochika; Nagao, Keisuke (4 April 2018). "Eruptive history of Incahuasi, Falso Azufre and El Cóndor Quaternary composite volcanoes, southern Central Andes". Bulletin of Volcanology. 80 (5): 20. Bibcode:2018BVol...80...44G. doi:10.1007/s00445-018-1221-5. ISSN 1432-0819. S2CID 134869390.
- 1 2 3 4 5 Richards & Villeneuve 2001, p. 79.
- 1 2 3 4 5 Gardeweg, Cornejo & Davidson 2010, p. 26.
- 1 2 Schroder & Makki 2000, p. 5.
- 1 2 Schröder & Schmidt 1997, p. 232.
- 1 2 Schröder & Schmidt 1997, p. 250.
- ↑ Schroder & Makki 2000, p. 27.
- 1 2 Gardeweg, Cornejo & Davidson 2010, p. 27.
- 1 2 3 4 5 6 7 8 9 10 11 Richards & Villeneuve 2001, p. 92.
- 1 2 3 Richards & Villeneuve 2001, p. 91.
- 1 2 Richards & Villeneuve 2002, p. 162.
- 1 2 3 Richards & Villeneuve 2002, p. 173.
- 1 2 Richards & Villeneuve 2001, p. 89,91.
- ↑ Richards, Jeremy P.; Ullrich, Thomas; Kerrich, Robert (April 2006). "The Late Miocene–Quaternary Antofalla volcanic complex, southern Puna, NW Argentina: Protracted history, diverse petrology, and economic potential". Journal of Volcanology and Geothermal Research. 152 (3–4): 198. Bibcode:2006JVGR..152..197R. doi:10.1016/j.jvolgeores.2005.10.006.
- ↑ Schröder & Schmidt 1997, p. 230,233.
- ↑ Gardeweg, Cornejo & Davidson 2010, p. 23.
- ↑ Vimercati et al. 2016, p. 587.
- ↑ Aran et al. 2021, p. 2295.
- ↑ Villalobos, Alvaro S.; Wiese, Jutta; Aguilar, Pablo; Dorador, Cristina; Imhoff, Johannes F. (1 June 2018). "Subtercola vilae sp. nov., a novel actinobacterium from an extremely high-altitude cold volcano lake in Chile". Antonie van Leeuwenhoek. 111 (6): 955–963. doi:10.1007/s10482-017-0994-4. ISSN 1572-9699. PMC 5945732. PMID 29214367.
- ↑ Kostka 2006, p. 57.
- 1 2 3 Richards & Villeneuve 2001, p. 101.
- 1 2 Richards & Villeneuve 2001, p. 95.
- ↑ Richards & Villeneuve 2001, p. 97.
- 1 2 Richards & Villeneuve 2001, p. 98.
- 1 2 Richards & Villeneuve 2001, p. 99.
- 1 2 Francis & Wells 1988, p. 269.
- ↑ Richards & Villeneuve 2001, p. 95,97.
- ↑ Francis & Wells 1988, p. 275.
- ↑ Richards & Villeneuve 2001, pp. 95–96.
- ↑ Francis & Wells 1988, p. 271.
- 1 2 Richards & Villeneuve 2001, p. 100.
- ↑ Richards & Villeneuve 2001, p. 102.
- ↑ "Volcán Llullaillaco". Glaciares de Chile (in Spanish). Laboratorio de Glacíologia. Retrieved 1 July 2021.
- ↑ Schroder & Makki 2000, p. 4.
- ↑ Hastenrath 1971, p. 257.
- ↑ Lliboutry, L.; González, O.; Simken, J. (1958). "Les glaciers du désert chilien". Extrait des Comptes Rendus et Rapports. Assemblee Generale de Toronto (in French). 4: 297.
- ↑ Messerli et al. 1992, p. 263.
- ↑ Grosjean, M.; Messerli, B.; Screier, H. (1991). "Seehochstände, Bodenbildung und Vergletscherung im Altiplano Nordchiles. Ein interdisziplinärer Beitrag zur Klimageschichte der Atacama. Erste Resultate" [Lake highstands, soil formation and glaciation in the Altiplano of Northern Chile. An interdisciplinary contribution to the climate history of the Atacama. First results.] (PDF). Bamberger Geographische Schriften (in German). 11: 104–105.
- 1 2 Vimercati et al. 2019, p. 191.
- ↑ Schroder & Makki 2000, p. 13.
- ↑ Lliboitry, Louis (1954-01-01). "The Origin of Penitents". Journal of Glaciology. 2 (15): 335. doi:10.3189/S0022143000025181. S2CID 129096835.
- ↑ Lynch, Thomas F. (April 1986). "Climate change and human settlement around the late-glacial laguna De Punta Negra, northern Chile: The preliminary results". Geoarchaeology. 1 (2): 148. doi:10.1002/gea.3340010203.
- ↑ Messerli et al. 1992, p. 257.
- ↑ Messerli et al. 1992, p. 262.
- ↑ Hastenrath 1971, p. 262.
- ↑ Schröder & Schmidt 1997, p. 251.
- 1 2 3 Schröder & Schmidt 1997, p. 253.
- ↑ Schröder & Schmidt 1997, p. 254.
- ↑ Schroder & Makki 2000, p. 33.
- 1 2 3 Schröder & Schmidt 1997, p. 246.
- ↑ Schroder & Makki 2000, p. 14,15.
- ↑ Schröder & Schmidt 1997, p. 252.
- ↑ Schroder & Makki 2000, p. 19.
- 1 2 Schröder & Schmidt 1997, p. 249.
- ↑ Schroder & Makki 2000, p. 12.
- 1 2 3 Richards & Villeneuve 2001, p. 93.
- ↑ Gardeweg, Cornejo & Davidson 2010, p. 35.
- 1 2 Richards & Villeneuve 2002, p. 178.
- ↑ Schröder & Schmidt 1997, p. 231.
- ↑ Richards & Villeneuve 2002, p. 192.
- ↑ Naranjo, José Antonio; Villa, Víctor; Ramírez, Cristián; Pérez de Arce, Carlos (26 January 2018). "Volcanism and tectonism in the southern Central Andes: Tempo, styles, and relationships". Geosphere. 14 (2): 636. Bibcode:2018Geosp..14..626N. doi:10.1130/GES01350.1. ISSN 1553-040X.
- ↑ Lazar 2005, p. 181.
- ↑ Schröder & Schmidt 1997, p. 239.
- ↑ Schröder & Schmidt 1997, p. 238.
- ↑ Schröder & Schmidt 1997, p. 236.
- 1 2 3 Schroder & Makki 2000, p. 9.
- ↑ Lazar 2005, p. 184.
- ↑ Schröder & Schmidt 1997, p. 235.
- 1 2 Schröder & Schmidt 1997, p. 234.
- ↑ Schroder & Makki 2000, p. 10.
- ↑ Storz, Jay F.; Quiroga-Carmona, Marcial; Opazo, Juan C.; Bowen, Thomas; Farson, Matthew; Steppan, Scott J.; D’Elía, Guillermo (4 August 2020). "Discovery of the world's highest-dwelling mammal". Proceedings of the National Academy of Sciences. 117 (31): 18169–18171. Bibcode:2020PNAS..11718169S. doi:10.1073/pnas.2005265117. ISSN 0027-8424. PMC 7414144. PMID 32675238.
- 1 2 3 Lynch, R. C.; King, A. J.; Farías, Mariá E.; Sowell, P.; Vitry, Christian; Schmidt, S. K. (June 2012). "The potential for microbial life in the highest-elevation (>6000 m.a.s.l.) mineral soils of the Atacama region". Journal of Geophysical Research: Biogeosciences. 117 (G2): n/a. Bibcode:2012JGRG..117.2028L. doi:10.1029/2012JG001961.
- ↑ Bull, Alan T.; Asenjo, Juan A.; Goodfellow, Michael; Gómez-Silva, Benito (8 September 2016). "The Atacama Desert: Technical Resources and the Growing Importance of Novel Microbial Diversity". Annual Review of Microbiology. 70 (1): 215–34. doi:10.1146/annurev-micro-102215-095236. PMID 27607552.
- ↑ Vimercati et al. 2019, p. 195.
- ↑ Vimercati et al. 2019, p. 197.
- ↑ Aran et al. 2021, p. 2296.
- ↑ Vimercati et al. 2016, p. 579.
- ↑ Vimercati et al. 2016, p. 586.
- ↑ Aran et al. 2021, p. 2297.
- ↑ Schröder & Schmidt 1997, p. 245.
- ↑ Fleetwood, Lachlan (5 October 2017). ""No former travellers having attained such a height on the Earth's surface": Instruments, inscriptions, and bodies in the Himalaya, 1800–1830". History of Science. 56 (1): 3–34. doi:10.1177/0073275317732254. PMID 28980481.
- 1 2 Mignone 2013, p. 145.
- 1 2 3 Previgliano et al. 2003, p. 1474.
- ↑ Reinhard, Johan; Ceruti, Maria Constanza (2009). Inca rituals and sacred mountains : a study of the world's highest archaeological site. Oakville: David Brown Book. ISBN 978-1-931745-77-2.
- ↑ Previgliano et al. 2003, p. 1475.
- ↑ Grady, Denise (2007-09-11). "In Argentina, a Museum Unveils a Long-Frozen Maiden". The New York Times. ISSN 0362-4331. Retrieved 2017-01-31.
- ↑ Giordano, Mariana (2016). "Performatividad, reliquia y visibilidad: Apariciones de "Lo Andino" en la fotografía indígena Argentina". Diálogo Andino (50): 07–19. doi:10.4067/S0719-26812016000200002. ISSN 0719-2681.
- 1 2 Mignone 2013, p. 148.
- ↑ Wilson, Andrew; Bradford, University of (2016-11-21). "Hair and Sacrifice in the Andean World, as deduced by biomolecular approaches". Internet Archaeology (42). doi:10.11141/ia.42.6.2. ISSN 1363-5387.
- ↑ Vitry, Christian (September 2020). "Los Caminos Ceremoniales en los Apus del Tawantinsuyu". Chungará (Arica). 52 (3): 509–521. doi:10.4067/S0717-73562020005001802. ISSN 0717-7356.
- ↑ Vitry 2016, p. 7.
- ↑ Vitry 2016, p. 6.
- ↑ Mignone 2013, pp. 147–148.
- ↑ Mignone 2013, p. 152.
- ↑ Vitry 2016, p. 3.
- ↑ Niedermann, S.; Althaus, T.; Hahne, K. (1998). "Dating of lava flows and landslides in the Chilean and Argentine Andes using cosmic-ray-produced 3He and ZINe" (PDF). Mineralogical Magazine. 62 (2): 1085–1086. Bibcode:1998MinM...62.1085N. doi:10.1180/minmag.1998.62A.2.234. Archived from the original (PDF) on 14 August 2017.
- ↑ Richards & Villeneuve 2001, p. 77.
- ↑ Rudolph, William E. (April 1955). "Licancabur: Mountain of the Atacamenos". Geographical Review. 45 (2): 151–171. doi:10.2307/212227. JSTOR 212227.
- 1 2 Amigo, Álvaro R.; Bertin, Daniel U.; Orozco, Gabriel L. (2012). Peligros volcánicos de la Zona Norte de Chile (PDF) (Report). Carta geológica de Chile: Serie Geología Ambiental (in Spanish). Vol. 17. SERVICIO NACIONAL DE GEOLOGÍA Y MINERÍA. p. 21. ISSN 0717-7305. Archived from the original (PDF) on June 29, 2021. Retrieved 20 August 2021.
- ↑ Solon, Adam J.; Vimercati, Lara; Darcy, J. L.; Arán, Pablo; Porazinska, Dorota; Dorador, C.; Farías, M. E.; Schmidt, S. K. (5 January 2018). "Microbial Communities of High-Elevation Fumaroles, Penitentes, and Dry Tephra "Soils" of the Puna de Atacama Volcanic Zone". Microbial Ecology. 76 (2): 340–351. doi:10.1007/s00248-017-1129-1. PMID 29305629. S2CID 1079993.
- ↑ Garcia, Sebastian; Badi, Gabriela (1 November 2021). "Towards the development of the first permanent volcano observatory in Argentina". Volcanica. 4 (S1): 26. doi:10.30909/vol.04.S1.2148. ISSN 2610-3540. S2CID 240436373.
Sources
- Aran, Pablo; Aguilar, Pablo; Bowen, Thomas; Farson, Matthew; Tapia, Joseline; Vimercati, Lara; Darcy, John L.; Solon, Adam J.; Porazinska, Dorota; Schmidt, Steven K.; Dorador, Cristina (2021). "Insights into an undescribed high-elevation lake (6,170 m a.s.l.) on Volcán Llullaillaco: A physical and microbiological view". Aquatic Conservation: Marine and Freshwater Ecosystems. 31 (8): 2293–2299. doi:10.1002/aqc.3612. ISSN 1099-0755. S2CID 236579383.
- Francis, P. W.; Wells, G. L. (July 1988). "Landsat Thematic Mapper observations of debris avalanche deposits in the Central Andes". Bulletin of Volcanology. 50 (4): 258–278. Bibcode:1988BVol...50..258F. doi:10.1007/BF01047488. S2CID 128824938.
- Gardeweg, Moyra M; Cornejo, Paula P; Davidson, John M (2010-08-30). "Geologia del Volcan Llullaillaco, Altiplan de Antofagasta, Chile (Andes Centrales)". Andean Geology (in Spanish) (23). ISSN 0718-7106.
- Hastenrath, Stefan L. (1971-01-01). "On the Pleistocene Snow-Line Depression in the Arid Regions of the South American Andes". Journal of Glaciology. 10 (59): 255–267. Bibcode:1971JGlac..10..255H. doi:10.3189/S0022143000013228.
- Kostka, Robert (2006). Aconcagua und die Anden bis zum Wendekreis des Steinbocks (in German). ISBN 978-3-7059-0229-9. OCLC 181499834.
- Lazar, Reinhold (April 2005). "Concept for a bioclimatic evaluation of an expedition and trekking area at moderate and high altitudes". Wiener Medizinische Wochenschrift (in German). 155 (7–8): 176–187. doi:10.1007/s10354-005-0167-3. PMID 15966264. S2CID 25806993.
- Messerli, Bruno; Grosjean, Martin; Graf, Kurt; Schotterer, Ueli; Schreier, Hans; Vuille, Mathias (1992). "Die Veränderungen von Klima und Umwelt in der Region Atacama (Nordchile) seit der Letzten Kaltzeit". Erdkunde (in German). 46 (3): 257–272. doi:10.3112/erdkunde.1992.03.09.
- Mignone, Pablo (2013-05-20). ""El camino hacia el Santuario Incaico del Llullaillaco": el complejo arqueológico más alto del mundo analizado a través de un SIG". Virtual Archaeology Review (in Spanish). 4 (8): 145–154. doi:10.4995/var.2013.4356. ISSN 1989-9947.
- Previgliano, Carlos H.; Ceruti, Constanza; Reinhard, Johan; Araoz, Facundo Arias; Diez, Josefina Gonzalez (December 2003). "Radiologic Evaluation of the Llullaillaco Mummies". American Journal of Roentgenology. 181 (6): 1473–1479. doi:10.2214/ajr.181.6.1811473. PMID 14627558.
- Richards, J.P.; Villeneuve, M. (January 2001). "The Llullaillaco volcano, northwest Argentina: construction by Pleistocene volcanism and destruction by sector collapse". Journal of Volcanology and Geothermal Research. 105 (1–2): 77–105. Bibcode:2001JVGR..105...77R. doi:10.1016/S0377-0273(00)00245-6.
- Richards, Jeremy P; Villeneuve, Michael (August 2002). "Characteristics of late Cenozoic volcanism along the Archibarca lineament from Cerro Llullaillaco to Corrida de Cori, northwest Argentina". Journal of Volcanology and Geothermal Research. 116 (3–4): 161–200. Bibcode:2002JVGR..116..161R. doi:10.1016/S0377-0273(01)00329-8.
- Schroder, H.; Makki, M. (2000-12-10). "Morfología periglacial del volcán Llullaillaco (Chile/Argentina)". Pirineos (in Spanish). 155: 3–36. doi:10.3989/pirineos.2000.v155.86. ISSN 1988-4281.
- Schröder, Hilmar; Schmidt, Dieter (1997). "Klimamorphologie und Morphogenese des Llullaillaco (Chile/Argentinien)". Mitteilungen der Fränkischen Geographischen Gesellschaft (in German). 44: 225–258. Retrieved 12 December 2016.
- Vimercati, L.; Hamsher, S.; Schubert, Z.; Schmidt, S. K. (17 June 2016). "Growth of high-elevation Cryptococcus sp. during extreme freeze–thaw cycles". Extremophiles. 20 (5): 579–588. doi:10.1007/s00792-016-0844-8. ISSN 1431-0651. PMID 27315166. S2CID 7269739.
- Vimercati, Lara; Solon, Adam J.; Krinsky, Alexandra; Arán, Pablo; Porazinska, Dorota L.; Darcy, John L.; Dorador, Cristina; Schmidt, Steven K. (1 January 2019). "Nieves penitentes are a new habitat for snow algae in one of the most extreme high-elevation environments on Earth". Arctic, Antarctic, and Alpine Research. 51 (1): 190–200. doi:10.1080/15230430.2019.1618115. ISSN 1523-0430.
- Vitry, Christian (2016). "Contribución al Estudio de Caminos se Sitios Arqueológicos de Altura. Volcán Llullaillaco (6.739 M). Salta – Argentina" (PDF). maam.gob.ar (in Spanish). Salta: Museo de Arqueologia de Alta Montana. Archived from the original (PDF) on 11 June 2016. Retrieved 12 December 2016Access date, not publication date
{{cite web}}
: CS1 maint: postscript (link)
Bibliography
- Darack, Ed (2001). Wild Winds: Adventures in the Highest Andes. Cordee / DPP. ISBN 978-1884980817.
- Reinhard, Johan: The Ice Maiden: Inca Mummies, Mountain Gods, and Sacred Sites in the Andes. National Geographic Society, Washington, D.C., 2005.
- Reinhard, Johan and Ceruti, María Constanza: "Inca Rituals and Sacred Mountains: A Study of the World's Highest Archaeological Sites" Los Angeles: UCLA, 2010.
- Reinhard, Johan and Ceruti, María Constanza: Investigaciones arqueológicas en el Volcán Llullaillaco: Complejo ceremonial incaico de alta montaña. Salta: EUCASA, 2000.
- Reinhard, Johan; Ceruti, María Constanza (2006). "Sacred Mountains, Ceremonial Sites and Human Sacrifice Among the Incas". Archaeoastronomy. 19: 1–43.
- Ceruti, María Constanza: Llullaillaco: Sacrificios y Ofrendas en un Santuario Inca de Alta Montaña. Salta: EUCASA, 2003.
- Reinhard, Johan (1993). "Llullaillaco: An Investigation of the World's Highest Archaeological Site". Latin American Indian Literatures Journal. 9 (1): 31–54.
- Beorchia, Antonio: "El cementerio indígena del volcán Llullaillaco." Revista del Centro de Investigaciones Arqueológicas de Alta Montaña 2: 36–42, 1975, San Juan.
- Previgliano, Carlos; Ceruti, Constanza; Reinhard, Johan; Arias, Facundo; Gonzalez, Josefina (2003). "Radiologic Evaluation of the Llullaillaco Mummies". American Journal of Roentgenology. 181 (6): 1473–1479. doi:10.2214/ajr.181.6.1811473. PMID 14627558.
- Wilson, Andrew; Taylor, Timothy; Ceruti, Constanza; Reinhard, Johan; Chávez, José Antonio; Grimes, Vaughan; Wolfram-Meier-Augenstein; Cartmell, Larry; Stern, Ben; Richards, Michael; Worobey, Michael; Barnes, Ian; Gilbert, Thomas (2007). "Stable isotope and DNA evidence for ritual sequences in Inca child sacrifice". Proceedings of the National Academy of Sciences. 104 (42): 16456–16461. Bibcode:2007PNAS..10416456W. doi:10.1073/pnas.0704276104. PMC 2034262. PMID 17923675.
- Complete description, history, place name and routes of Llullaillaco in Andeshandbook
- Museum of High Mountain Archaeology Archived 2013-10-04 at the Wayback Machine (in Spanish)
- "Llullaillaco". SummitPost.org.
External links

Reise durch die Wüste Atacama, auf Befehl der Chilenischen Regierung im Sommer 1853–54 unternommen. Anton. 1860.