TRPV6 | |||||||||||||||||||||||||||||||||||||||||||||||||||
---|---|---|---|---|---|---|---|---|---|---|---|---|---|---|---|---|---|---|---|---|---|---|---|---|---|---|---|---|---|---|---|---|---|---|---|---|---|---|---|---|---|---|---|---|---|---|---|---|---|---|---|
| |||||||||||||||||||||||||||||||||||||||||||||||||||
Identifiers | |||||||||||||||||||||||||||||||||||||||||||||||||||
Aliases | TRPV6, ABP/ZF, CAT1, CATL, ECAC2, HSA277909, LP6728, ZFAB, transient receptor potential cation channel subfamily V member 6, HRPTTN | ||||||||||||||||||||||||||||||||||||||||||||||||||
External IDs | OMIM: 606680 MGI: 1927259 HomoloGene: 56812 GeneCards: TRPV6 | ||||||||||||||||||||||||||||||||||||||||||||||||||
| |||||||||||||||||||||||||||||||||||||||||||||||||||
| |||||||||||||||||||||||||||||||||||||||||||||||||||
| |||||||||||||||||||||||||||||||||||||||||||||||||||
| |||||||||||||||||||||||||||||||||||||||||||||||||||
| |||||||||||||||||||||||||||||||||||||||||||||||||||
Wikidata | |||||||||||||||||||||||||||||||||||||||||||||||||||
|
TRPV6 is a membrane calcium (Ca2+) channel protein which is particularly involved in the first step in Ca2+absorption in the intestine.
Classification
Transient Receptor Potential Vanilloid subfamily member 6 (TRPV6) is an epithelial Ca2+ channel that belongs to the transient receptor potential family (TRP) of proteins.[5] The TRP family is a group of channel proteins critical for ionic homeostasis and the perception of various physical and chemical stimuli. TRP channels can detect temperature, osmotic pressure, olfaction, taste, and mechanical forces.[5][6] The human genome encodes for 28 TRP channels, which include six TRPV channels.[5] The high Ca2+-selectivity of TRPV5 and TRPV6 makes these channels distinct from the other four TRPV channels (TRPV1-TRPV4).[7] TRPV5 and TRPV6 are involved in Ca2+ transport, whereas TRPV1 through TRPV3 are heat sensors with different temperature threshold for activation, and TRPV4 is involved in sensing osmolarity.[8][9] Genetic defects in TRPV6 gene are linked to transient neonatal hyperparathyroidism and early-onset chronic pancreatitis. Dysregulation of TRPV6 is also involved in hypercalciuria, kidney stone formation, bone disorders, defects in keratinocyte differentiation, skeletal deformities, osteoarthritis, male sterility, Pendred syndrome, and certain sub-types of Cancer.[8][9]
Identification
Peng et al identified TRPV6 in 1999 from rat duodenum in an effort to search for Ca2+ transporting proteins involved in Ca2+absorption.[10] TRPV6 was also called calcium transport protein 1 (CaT1)[10][11] initially although the names epithelial calcium channel 2 (ECaC2)[12][13] and CaT1-like (CaT-L)[14] were also used in early studies to describe the channel.[10][12][13][14] The human and mouse orthologs of TRPV6 were cloned by Peng et al and Weber et al, respectively.[11][12] The name TRPV6 was confirmed in 2005.[15]
Gene location, chromosomal location, and phylogeny
The human TRPV6 gene is located on chromosomal locus 7q33-34 close to its homolog TRPV5 on 7q35.[16][17] The TRPV6 gene in human encodes for 2906 bp-long mRNA.[17] In contrast to most other proteins, which initiate translation with an AUG codon, TRPV6 translation is initiated by non-AUG-codon-mediated reading.[18] TRPV6 protein bears a 40-a.a-long N-terminal extension in placenta and in some physiological settings in comparison to the annotated version of the protein used in biological studies.[18] However, it is still to be determined whether the long version of the TRPV6 protein is the dominant form in different tissues.
Species | Human | Rat | Mouse |
Chromosomal location | 7q33-q34 | 4q22 | 6B2 |
Annotated aa length | 725 | 727 | 727 |
In vivo aa lengtha | 765 | 767 | 767 |
RefSeq nucleotide | NM_018646 | NM_053686 | NM_022413 |
RefSeq protein | NP_061116 | NP_446138 | NP_071858 |
aTo be verified in different tissues.
It has been hypothesized that Trpv5 and Trpv6 genes were generated from a single ancestral gene by gene duplication events.[16][19] Phylogenetic analysis has shown that TRPV6 paralogs in mammals, sauropsids, amphibians, and chondrichthyes arose out of independent duplication events in the ancestor of each group.[19] It is speculated that two specialized Ca2+-selective Trpv homologs arose as an adaptation to achieve a greater degree of functional specialization for navigating distinct renal challenges of terrestrial animals.[19]
Two alleles of the TRPV6 gene have been identified in humans (originally noted as CaT-La and CaT-Lb).[14] These alleles exhibit coupled polymorphisms generating two versions of the same gene.[14][20] The polymorphisms give rise to an “ancestral variant” and a “derived variant” that differ in five bases and three amino acids.[14][20] The ancestral allele codes for C197(157, in parentheses are annotated amino acid numbering), M418(378), and M721(681) whereas the derived allele codes for R197(157), V418 (378) and T721(681).[20][21] The frequency of the ancestral TRPV6 allele varies across different population groups.[20][21] It is hypothesized that selection pressures that could have changed TRPV6 allele distribution include changes in patterns of milk consumption, domestication of animals, change in ultraviolet light exposure due to trans-equatorial migration, genomic adaptations providing immune advantages to populations encountering new pathogens.[20][21][22]
Tissue distribution
The TRPV6 protein is expressed in epithelial tissues such as the intestine, kidney, placenta, epididymis, and exocrine glands such as pancreas, prostate and salivary, sweat, and mammary glands.[23][24] TRPV6 protein expression in humans has been demonstrated in the esophagus, stomach, small intestine, colon, pancreas, mammary glands, ovary, thyroid, and prostate by immunohistochemistry approaches.[23] TRPV6 expression mainly confines on the apical membrane of epithelial cells. In the intestine, the protein is expressed on the brush-border membrane of enterocyte.
Differences in the TRPV6 expression profile have been reported possibly due to variation in assay-dependent such primer design, hybridization probes, PCR vs. northern blotting, semi-quantitative PCR vs. RT-PCR, and antibodies used for immunodetection.[25] TRPV6 expression profile is also influenced by age, gender, Ca2+ and vitamin D3 levels in food, hormonal status, location within the tissue, cellular location, reproductive status, and weaning status (see Section Regulation).
In humans, TRPV6 transcripts have been detected in the placenta, pancreas, prostate cancer, and duodenum and the prostate by northern blotting; and in duodenum, jejunum, placenta, pancreas, testis, kidney, brain, and colon by semi-quantitative PCR.[13] In rodents, TRPV6 expression has been validated in the duodenum, cecum, small intestine, colon, placenta, pancreas, prostate, and epididymis by Northern Blotting.[10][17][26] In mouse, TRPV6 transcript abundance measured by RT-PCR is as follows: prostate > stomach, brain > lung > duodenum, cecum, heart, kidney, bone > colon > skeletal muscle > pancreas.[27]
Data from Human Protein Atlas and RNA-Seq based suggest TRPV6 mRNA is low in most tissues except for the placenta, salivary gland, pancreas, and prostate.[24][28] TRPV6 mRNA is expressed in the apical domain of murine osteoclasts of cortical bone.[29][30] Cortical and trabecular osteocytes do not express TRPV6 mRNA whereas osteoblasts show weak expression.[31]
Structure and biophysical properties
Primary and secondary structure
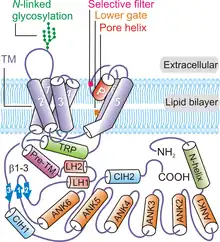
Overall, four subunits of TRPV6 arrange to form a tetrameric channel displaying a four-fold symmetry.[8][32] Beginning from N-terminus and moving towards the C-terminus of the protein, each TRPV6 polypeptide contains: an N-terminal helix, an ankyrin repeat domain (ARD) containing six ankyrin repeats, a β-hairpin structure linker domain made up two β-strands, a helix-turn-helix motif, a pre-SI helix, TM domain made up of six TM helices (S1 through S6), a pore-loop (also called P-loop), amphipathic TRP helix, C-terminal hook, and a six-residue β-strand (β3) (Figure 1).[8][32]
Tertiary and quaternary structure
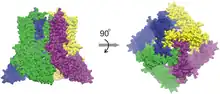
The TRPV6 channel protein displays four-fold symmetry and contains two main compartments: a 30 Å-tall transmembrane domain with a central ion channel pore and a ~70 Å-tall and a ~110 Å-wide intracellular skirt enclosing a 50 Å × 50 Å cavity wide cavity underneath the ion channel.[32] The clustering of four TRPV6 subunits forms an aqueous pore exhibiting a fourfold symmetry (Figure 2). A pre-SI helix links the intracellular portion of the protein to the TM domain through a linker domain made up of β-hairpin structure and a helix-turn-helix motif. Helices S1 through S4 form a transmembrane helical bundle or TM domain that is inserted almost perpendicularly to the plane of the plasma membrane.[32]
The pore module elements are made up of S5, S6, and the P-loop in TM domains.[32] The pore module from each TRPV6 polypeptide participates in inter-subunit interactions to form a central ion pore (Figure 1).[32] The pore-forming elements of each TRPV6 subunit also interact with S1-S4 domains of the adjacent polypeptide in a domain-swapped arrangement.[32][33] Intersubunit interactions also occur between S1-S2 extracellular loops and S5-P and S6-P loops of the neighboring TRPV6 subunits.[32] The conserved N-linked glycosylation site on the S1-S2 loop is required for by the Klotho-mediated activation.[34] The intracellular skirt portion of the TRPV6 protein is mainly made up of the ankyrin repeats.[32] The TRP domain is oriented parallel to the membrane and participates in hydrophobic interactions with the TM domain and the hydrophilic interactions in the intracellular skirt. The N-terminal helix, C-terminal hook, and β-sheets (formed by the β-hairpin structure in the linker domain) in the channel participates in intersubunit interactions with the ARDs to provides a framework for holding the elements of the intracellular skirt together.[8][32]
Pore architecture and cation binding sites
The TRPV6 pore has four main elements, namely, the extracellular vestibule, a selectivity filter, a hydrophobic cavity, and a lower gate.[32][35][36] Facing the central lumen of the channel, a four-residue selectivity filter (538TIID541) containing four Aspartate 541 (D541) side chains (one from each protomer) is critical for Ca2+ selectivity and other biophysical properties of the channel.[32][35][36] This filter forms a negatively charged ring that discriminates between ions based on their size and charge. Mutations in the critical pore-forming residue of TRPV6 blocks Ca2+uptake, a strategy has been used to generate TRPV6 loss-of-function models to examine the role of the channel in animal physiology.[35][36] Four different types of cation binding sites are thought to exist in the TRPV6 channel.[32] Site 1 is located in the central pore and shares the same plane that is occupied by the key selective residues D541. Site 2 is thought to be present about 6-8 Å below Site 1 followed by Site 3 which is located in the central pore axis about 6.8 Å below Site 2. Site 2 and 3 are thought to interact with partially-hydrated to equatorially-hydrated Ca2+ ions. Finally, four symmetrical cation binding sites in the extracellular vestibule mediate the recruitment of cations towards the extracellular vestibule of TRPV6 and are referred to as recruitment sites.[32]
Ion permeation
The conductance of TRPV6 for divalent cations follows the preference: Ca2+ > Ba2+ > Sr2+ > Mn2. Intra-cellular Mg2+ inhibits TRPV6 and contributes to the strong inward rectification exhibited by the channel.[37] TRPV6 uptake activity is inhibited by divalent Pb2, Cu2+, Cd2+, Zn2, Co2+, Fe2+, and trivalent cations La3+, Fe3+, Gd3+. The concentration of ions to achieve the inhibition ranges from 1 to 10 μM.[38] The TRPV6 protein is constitutive with a single-channel conductance of 42-58 ps.[7][39] At low Ca2+ concentrations, a single Ca2+ ion binds in the selectivity filter formed by D541 and permits Na+ permeation. At high Ca2+ concentration, Ca2+ permeation occurs by a knock-off mechanism that involves the formation of short-lived conformations involving binding of three Ca2+ ions to residue D541.[39]
Channel gating
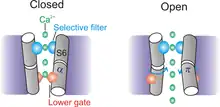
The conformational changes involved in channel opening are hinged around the residue Alanine 566 (A566) and occur in the pore-lining helix S6 (Figure 3).[39] The upper portion of S6 helix undergoes an α-to-π helical transition which forces the lower portion of the helix to turn by 100 degrees and tilt away from the pore axis by 11 degrees.[39] This conformational change moves the lower portion of the helix gating the pore and thereby widens the pore size. The conformational change alters the residues facing the pore axis and triggers the formation of new electrostatic bonds subunit and salt bridges that offset the high energetic cost of unfavorable α-to-π helical transition that occurs during channel opening.[39]
Regulation by phosphatidylinositol 4,5-bisphosphate (PIP2) and calmodulin (CaM)
The influx of Ca2+ inside the cell triggers negative feedback mechanisms to suppress TRPV6 activity and prevent Ca2+ overload.[9] TRPV6 channel activity is regulated by the intracellular level of phospholipid phosphatidylinositol 4,5-bisphosphate (PIP2) and interactions with Ca2+-Calmodulin (CaM) complex.[9] The depletion of PIP2 or CaM-binding inactivates TRPV6.[40][41][42][43][44] The influx of Ca2+ in TRPV6 expressing cells activates phospholipase C (PLC) which in turn hydrolyzes PIP2. Depletion in PIP2 levels results in a decline in channel activity since most TRP channels require this lipid for activation.[40][43][44] The lipid PIP2 can override Ca2+-CaM-mediated inhibition of TRPV6. Overall, TRPV6 inactivation by calmodulin is orchestrated by a balance of intracellular Ca2+ and PIP2 concentration.[40][41][42][43][44]
Interacting proteins
Among 20+ TRPV6 interactors identified so far, the functional consequences of Ca2+-binding protein Calmodulin (CaM) and Glucuronidase Klotho have been most extensively characterized [36, 37, 41, 42].[34][40][41][45][46] Functional consequences of TRPV6 channel activation are summarized in the table below).[47]
Interactor | Consequence |
BSPRY | N/A |
Calbindin-D28k | N/A |
Calmodulin | Inhibition |
Cyclophilin B | Activation |
FYN | PO4lyation |
I-MFA | N/A |
Klotho | Activation, Glycosylation (Asn-357) |
NHERF4 | Activation |
NIPSNAP1 | Inhibition |
NUMB | Inhibition |
PTEN | N/A |
PTP1B | DePO4lyation
(Tyr-161 and Tyr-162) |
RAB11A | Activation,
Increase in Plasma membrane level |
RGS2 | N/A |
RYR1 | N/A |
S100A10 | Activation,
Increase in Plasma membrane level |
SRC | PO4lyation (Tyr-161, 162) |
TRPC1 | Retains in ER, Inhibition |
TRPML3 | N/A |
TRPV5 | Tetramer formation,
New Channel creation |
Abbreviations
Protein Interactor
BSPRY: B-Box and Spry Domain Containing Protein; FYN: Fyn Kinase Belonging Src Family of Kinases; I-MFA: Myo D Family Inhibitor; NHERF: Na Exchanger Regulatory Factor; NIPSNAP14-Nitrophenylphosphatase Domain and Non-Neuronal SNAP25-Like Protein Homolog 1; Numb: Drosophila mutation that removes most of the sensory neurons in the developing peripheral nervous system; PTP: Protein Tyrosine Phosphatase; Rab11a: Member RAS Oncogene Family; RGS2: Regulator Of G-Protein Signaling 2; RyR1: Ryanodine Receptor 1; TRPC1: Transient receptor potential canonical 1; TRPML3: Transient receptor potential Mucolipin-3.
Physiological functions
The Ca2+-selective channel proteins TRPV6 and TRPV5 cooperate to maintain calcium concentration in specific organs.[22][48] TRPV6 functions as apical Ca2+ entry channels mediating transcellular transport of this ion in the intestine, placenta, and possibly some other exocrine organs. TRPV6 also plays important roles in maternal-fetal calcium transport,[49] keratinocyte differentiation,[50] and Ca2+ homeostasis in the endolymphatic system of the vestibular system,[51][52] and maintenance of male fertility.[53][54]
Ca2+ absorption in intestine
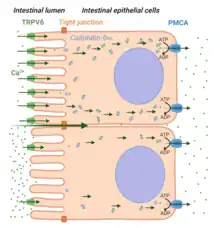
Two routes of Ca2+ absorption are recognized: paracellular transport and transcellular transport (see Figure 4).[55] A high-Ca2+-diet favors paracellular transport of the ion across the length of the intestine allowing them to pass between the intercellular tight junctions that connect epithelial cells. In contrast under conditions when [Ca2+] in the lumen of the intestine is lower in comparison to its concentration in the plasma (e.g. during low dietary Ca2+), the transcellular pathway is required for adequate Ca2+ absorption. Three important steps in transcellular Ca2+ transport are recognized: cellular entry of Ca2+ ion on the apical side via TRPV6 (Step-1), the binding of Ca2+ ion with calbindin-D9k (Step-2), and exit of Ca2+ from the basolateral side via the plasma membrane Ca2+ ATPase (PMCA1b).[55] The hormone Vitamin D3 (or 1,25(OH)2D3) plays an important role in TRPV6-mediated intestinal Ca2+ absorption).[55]
Ca2+ reabsorption in the kidney
In contrast to the intestine, where TRPV6 is the gatekeeper of Ca2+ absorption, the transcellular reabsorption of this ion in the kidney occurs through TRPV5. Although TRPV5 is a recognized gatekeeper for transcellular reabsorption of Ca2+ ion in the kidney, TRPV6 knockout (KO) mice also struggle to concentrate their urine and display hypercalciuria.[56] TRPV6 is known to co-localize with TRPV5 Calbindin-D28K in apical domains of distal convoluted tubules and connecting tubules [20]. TRPV5 KO mice compensate for Ca2+ loss by increasing TRPV6 expression in the duodenum.[56] Moreover, a recent study analyzing vitamin D responsive genes in ovine, canine and, equine kidney suggested that TRPV6, calD9k/calD28k, and PMCA could be the main pathways orchestrating transcellular Ca2+ transport in the kidney of sheep, dogs, and horses.[57]
Maternal-fetal Ca2+ transport
TRPV6 plays an indispensable role in placental Ca2+ transport.[49] Fetal bone mineralization peaks during late pregnancy. At this stage, fetal blood has a higher concentration of Ca2+ in comparison to maternal blood thereby creating conditions that require active transcellular transport of Ca2+ from mother to the fetus.[58][59] This process is very important since defects in placental transport of calcium can be precursors for Ca2+ deficiency syndromes and intrauterine growth restrictions.[60] The expression of TRPV6 increases 14-fold during the last 4 days of the murine gestational period and coincides with the peak phase of fetal bone mineralization.[49] The protein TRPV6 is abundantly expressed in the mammalian placental tissues.[49][61][62][63][64] Indeed, TRPV6 expression is ~1000-fold higher in comparison to TRPV5. In the placenta, TRPV6 is expressed in trophoblasts and syncytiotrophoblasts.[14][61] In mice, TRPV6 mRNA and protein are expressed in the intraplacental yolk sac and the visceral layer of the extraplacental yolk sac.[49] Most importantly, TRPV6 KO fetuses exhibit a 40% reduction in 45Ca2+ transport activity and a dramatic decrease in the ash weight (a measure of fetal bone health).[49] In humans, trophoblasts fluid shear stress (FSS) is known to induce a TRPV6-mediated Ca2+ influx and promote microvilli formation through a mechanism involving Ezrin and Akt-phosphorylation.[65]
Epididymal Ca2+ regulation and implications on male fertility
The regulation of calcium concentration in the epididymal lumen is critical for sperm motility.[66] TRPV6-mediated reduction of luminal Ca2+ concentration in the epididymis is critical for male fertility in mice.[53] TRPV6 KO mice or mice expressing loss-of-function version of TRPV6 channel (Trpv6D541A homozygous mice) have a severely impaired fertility.[53] Mice expressing nonfunctional TRPV6 have a 10-fold higher concentration of Ca2+ in the epididymal lumen and Ca2+ uptake in this space is reduced by 7-to-8 folds.[53][54] The increases Ca2+ ion in epididymal lumen concentration leads to significant defects in motility, fertilization capacity, and viability of sperms in TRPV6D541A mice.[53][54] It appears TRPV6 and chloride channel transmembrane manner 16 A (TMEM16A) act cooperatively to reduce the luminal concentration of Ca2+ in the epididymal lumen.[67]
Bone health
Under conditions of sub-optimal dietary Ca2+, normal serum calcium levels in TRPV6 KO mice are maintained at the expense of bone.[68][69] TRPV6 plays an important role in osteoclasts but not in osteoblasts.[68][69] In mice, TRPV6 depletion results in increased osteoclasts differentiation[29] whereas TRPV5 is essential for proper osteoclastic bone resorption.[68]
Keratinocyte differentiation
Keratinocytes differentiation is orchestrated by calcium switch, a process that entails an influx of Ca2+ in keratinocyte which induces broad transcriptional changes necessary for desmosome formation, stratification, and cornification.[70] TRPV6 KO mice display thinner layers of stratum corneum and 20% of the mice also show alopecia and dermatitis.[56] The silencing of TRPV6 impairs Ca2+-mediated differentiation of human primary keratinocytes and downregulates differentiation markers such as involucrin, transglutaminase-1, and cytokeratin-10. The hormone 1,25-dihydroxyvitamin-D3 upregulates TRPV6 in keratinocytes and triggers a Ca2+ influx. This in turn induces the expression of keratinocyte differentiation-specific pathways.[50]
Role in the inner ear
The proteins TRPV5 and TRPV6 are expressed in several regions of the inner ear as well as in primary cultures of semicircular canal duct (SCCD) epithelium.[51][52] Some studies have indicated that TRPV5 and TRPV6 are needed for lowering the Ca2+ concentration in the lumen of mammalian endolymph, a requirement that is essential for normal hearing and balance.[51][52][71]
Uterine and placental expression of TRPV6 and implications in pregnancy
The endometrial and uterine expression of TRPV6 has been reported in mammals.[72][73][74] The expression of TRPV6 in the uterus is thought to be hormonally regulated by 17β-estradiol and progesterone in rodents. In rodents, TRPV6 mRNA is expressed in the labyrinth and spongy zone as well as placenta-unattached areas of the uterus. The stage of pregnancy is an important regulator of TRPV6 expression. The downregulation of TRPV5/6 expression and a resulting decline in Ca2+ transport is thought to change the proliferative profile of human trophoblasts; a process which in turn is linked to the development of pre-eclampsia.[73] This juxtaposition of TRPV6 expression and its stringent regulation by sex hormones during pregnancy suggest that the protein may be important for embryo implantation, however conclusive evidence for this connection does not exist.[72][73][74]
Implications in Human Diseases
Transient Neonatal Hyperparathyroidism
Loss of TRPV6 in murine placenta severely impairs Ca2+ transport across trophoblast and reduces embryo growth, induces bone calcification, and impairs bone development. In humans, the insufficient maternal-fetal transport caused by pathogenic genomic variants of TRPV6 is thought to be a cause for skeletal defects observed in selected case reports of transient neonatal hyperparathyroidism (TNHP) cases. These variants are believed to compromise the plasma membrane localization of the protein. Exome sequencing of an infant with severe antenatal onset thoracic insufficiency with accompanying fetal skeletal abnormalities indicates the critical role of TRPV6 in maternal-fetal transport. The study indicated that compound heterozygous variants of TRPV6 result in severe undermineralization and severe dysplasia of the fetal skeleton.[75][76][77]
Chronic Pancreatitis
Recent evidence indicates that naturally occurring TRPV6 loss of function variants predisposes certain demographics to chronic pancreatitis (CP) by dysregulating calcium homeostasis in the pancreatic cells.[78][79] Sequencing studies among chronic pancreatitis patients revealed the presence of 33 missense and 2 nonsense variants predisposed Japanese, German, and French patients to a higher risk of CP.[79] Overall, these studies have shown that disease-inducing TRPV6 loss-of-function genomic variants are over-represented in German, French, Chinese, and Japanese CP patients in comparison to controls in their respective groups.[78][79] The loss-of-function variants are believed to compromise calcium transport in the pancreas by act by either reducing the total protein level and/or compromising Ca2+ uptake activity by the channel.[79]
Kidney Stone Formation
The role of TRPV6 in renal stone formation has been suggested through sequencing studies conducted on a cohort of 170 patients in Switzerland.[80] The studies revealed that the frequency of TRPV6 gain-of-function haplotype is significantly higher in Ca2+-stone formers (nephrolithiasis) in comparison to non-formers. The observed hypercalciuria phenotypes from animal studies and studies on TRPV6 single nucleotide polymorphisms (SNPs) suggest that TRPV6 haplotype could be an important risk factor for absorptive and renal hypercalciuria (kidney stones due to impaired intestinal absorption and renal re-absorption respectively). The lower incidence of kidney stone diseases in African-Americans and a relatively higher prevalence of ancestral haplotype suggest theory according to which this haplotype endows an advantage of increased Ca2+ reabsorption in this demographic and reduces the incidence of kidney stones.[14][20][22][80]
Bone Resorptive Diseases
TRPV6 KO mice exhibit osteoporosis-like symptoms such as reduced bone mineral density and hypercalciuria.[56] The hormone estrogen, the deficiency of which is linked to post-menopausal osteoporosis, also regulates the expression of TRPV6 in humans. Indeed, a lower calcium absorption seen in older postmenopausal women is attributed to reduced TRPV6.[81] The C-terminal portion of Soricidin is a drug that inhibits Ca2+-uptake activity by binding to TRPV6. Preclinical studies of this drug have shown great promise in the treatment of bone resorptive diseases.[28]
The high degree of similarity between Hereditary Vitamin D–Resistant Rickets (HVDRR) disease symptoms and observed phenotypes in TRPV6 KO mice has led some experts to postulate pathological connections between the disease and TRPV6 dysfunction.[48] TRPV6 plays an important chondroprotective role by regulating multiple aspects of chondrocyte function, such as extracellular matrix secretion, the release of matrix-degrading enzymes, cell proliferation, and apoptosis.[82] Furthermore, TRPV6 knockout mice display multiple osteoarthritis (OA) phenotypes such as cartilage fibrillation, eburnation, and loss of proteoglycans.[82]
Pendred Syndrome
The dysfunction gene Slc26a4 has been linked to Pendred syndrome – a genetic disorder that results in syndromic deafness in children.[71][83] The disease is caused by mutations in which compromise the function of the encoded protein pendrin - an anion Cl−/HCO3 − exchanger expressed in the inner ear.[71][83] The loss of function in this gene is thought to reduce the pH value of mammalian endolymph and impair Ca2+ absorption via TRPV5 and TRPV6.[83] This in turn could prevent the uptake of Ca2+ and impairs the luminal reduction in Ca2+ concentration within the endolymphatic system of the ear.[71][83]
Cancer
The overexpression of TRPV6 has been validated in the colon, parathyroid, pancreatic, and thyroid cancer[23] whereas its expression is reportedly downregulated in esophageal cancer,[84] non-small cell lung cancer,[85] and renal cancer.[86] TRPV6 is considered to be an oncochannel that is hypothesized to mediate cancer progression by triggering Ca2+-entry induced aberrations in molecular drivers regulating processes such as cell cycle, apoptosis, and migration; thereby conferring proliferative and survival advantages to cancer cells.[25][28][87] Overexpression of TRPV6 correlates strongly with pathological stage, tumor grade, extra-prostatic invasion, lymph node metastasis, and resistance to androgen-targeted therapies in prostate cancer.[14][23][87][88] The expression TRPV6 has been touted as a prognostic marker for advanced prostate cancer since its expression is strongly dependent on the grade of the tumor.[87][88] Expression of TRPV6 is significantly elevated in breast adenocarcinoma tissue in comparison to normal breast tissue.[89][90] TRPV6 expression has been reported multiple breast cancer cell lines and prostate cancer cell lines.[87][91][92][93] The prostate cancer cell lines PC-3 and LnCAP overexpress TRPV6 relative to benign epithelial cells PrEC and BPH-1.[87] The silencing of TRPV6 in prostate cancer cells decreases proliferation rate, S-phase accumulation, and expression of tumor marker proliferating cell nuclear antigen (PCNA) expression.[92] TRPV6 overexpression is believed to induce aberrant Ca2+-uptake in prostate cancer line and activate transcription factor Nuclear Factor of Activated T cells (NFAT).[92]
Expression of TRPV6 is upregulated by estrogen, progesterone, and estradiol in breast cancer cell line T47D.[90] In agreement with these observations, the estrogen receptor antagonist Tamoxifen reduces TRPV6 expression in T47D cells and suppresses Ca2+-uptake of the channel in both ER-positive and ER-negative breast cancer cell lines.[94] The overexpression of TRPV6 is associated with early-stage colon cancer and its silencing in colon cancer induces apoptosis and inhibits cancer cell proliferation.[95] In terms of mechanism, mutations within the calmodulin-binding domains of TRPV6 channels confers invasive properties to colon adenocarcinoma cells.[96] The proteins p38α and GADD45α are believed to upregulate TRPV6 expression signaling in SW480 colon cancer cells by enhancing vitamin D signaling.[97] TRPV6 has been reported to amplify Insulin-like growth factors (IGF)-induced PI3K-PDK1-Akt signaling in human colon cancer and promote colon cancer.[98]
TRPV6 is up-regulated in primary cancer tissues from pancreatic cancer patients and promotes the proliferation of pancreatic neuroendocrine tumors NFAT-dependent mechanisms.[99] Silencing of TRPV6 induces apoptosis and cell cycle arrest in pancreatic cancer cells and inhibits their invasion, proliferation, and migration.[100] Forced expression of TRPV6 in gastric cancer cells increases their sensitivity to capsaicin-induced apoptosis whereas the siRNA-mediated silencing of the channel suppresses this sensitivity.[101] TRPV6 downregulation in esophageal carcinoma has been suggested to be a prognostic marker of disease-specific survival in patients suffering from esophageal cancer.[102] Low TRPV5 and TRPV6 co-expression have suggested as predictive markers for poor recurrence-free survival in non-small cell lung cancer.[85]
Pharmacological Targeting
Several chemical inhibitors are known to inhibit TRPV6. Some compounds that have demonstrated inhibitory activity towards TRPV6 include TH-1177, 2-Aminoethoxydiphenyl borate (2-APB), 2-APB derivative 22b, Econazole, Miconazole, Piperazine derivative Cis-22a, Capsaicin, Δ9-tetrahydrocannabivarin, Xestospongin C, Lidocaine, gold-caged nanoparticle (PTX-PP@Au NPs) and Sorcidin C-13 (SOR-C13) synthetic peptide.[28] Among different inhibition strategies tested so far, the 13-amino acid peptide SOR-C13 has shown the most promise. This 13-amino acid peptide derived from 54-amino acid peptide found in the paralytic venom of the northern short-tailed shrew (Blarina brevicauda) reduces cancer growth in cell and animal models. This anti-cancer agent has recently completed a Phase I clinical safety trial that had enrolled 23 patients with advanced solid tumors of epithelial origin non-responsive to all standard-of-care treatments.[28]
Regulation
The regulation of TRPV6 can be examined mainly in the context of its physiological, hormonal, and molecular factors.[22] The hormonal regulation of TRPV6 has been characterized most extensively. In this regard, its regulation by the hormone vitamin D3 and sex hormones has been examined in considerable detail. Rodent studies suggest that the TRPV6 channel is regulated by a wide range of physiological factors such as diet, age, gender, pregnancy, lactation, sex hormones, exercise, age, and gender. Some biological and pharmacological agents known to regulate TRPV6 include glucocorticoids, immunosuppressive drugs, and diuretics.[22]
Vitamin D
Multiple dose-response and time-course experiments in rodents and colon cancer cell lines have conclusively shown TRPV6 mRNA is robustly induced by this vitamin D at extremely low concentrations.[103][104] At least five vitamin D response elements (VDREs) at positions −1.2, −2.1, −3.5, −4.3, and −5.5 kb relative to transcriptional start site (TSS) have been identified on TRPV6 transcripts.[105] Among these five sites, VDREs at positions −1.2, −2.1, and −4.3 kb are significantly more responsive to 1,25-(OH)2D3 in comparison to VDREs located at −3.5 and −5.5 kb which do not appear to contribute substantially to vitamin D mediated transcriptional regulation in the intestine.[105] Mechanism wise, TRPV6 transcription is initiated in response to vitamin D Receptor (VDR)-mediated signaling, although other non-direct mechanisms cannot be ruled out.[104][106] Important steps in vitamin D mediated transcriptional regulation include 1) binding of vitamin D on its cognate vitamin D receptor (VDR), 2) the translocation of vitamin D receptor (VDR)-retinoid X receptor heterodimer complex in the nucleus, 3) binding VDR-RXR complex on the TRPV6 gene promoter, 4) recruitment of steroid receptor coactivator 1 and RNA polymerase II on the promoter, and 5) transcriptional activation mediated through histone H4 acetylation events.[107]
Diet
The level of Ca2+ and vitamin D in the diet are the most important regulators of TRPV6 expression.[104] The expression of TRPV6 is thought to be modulated strongly to fine-tune Ca2+ absorption from the diet, especially under conditions when dietary Ca2+ availability is low.[103][104] In rodents, restricting Ca2+ availability in the diet induces dramatic up-regulation in the duodenal expression of TRPV6.[103][104] Calcium influx from the diet and its subsequent binding to calbindin-D9k could be the rate-limiting step that modulates vitamin D-dependent regulation TRPV6.[108] When dietary Ca2+ is insufficient, normal blood calcium levels in TRPV6 KO mice are maintained at the expense of bone.[68][69] In many rodent lines, genetic variations in TRPV6, calbindin-D9k, PMCA1b mRNA influence intestinal Ca absorption and its impact on bone marrow density.[109]
Pregnancy and lactation
Duodenal expression of TRPV6 transcripts is upregulated in WT and VDR KO mice during pregnancy and lactation.[110] The hormone prolactin upregulates TRPV6 transcription and facilitates an increase in intestinal Ca2+ absorption in lactating and pregnant rats, possibly as an adaptive mechanism for overcoming the loss in bone mineralization content during lactation.[111]
Aging
The intestinal expression of TRPV6 in mice varies dramatically by age and relative tissue location.[112] The duodenal expression of TRPV6 is undetectable at P1 and increases 6-fold as mice age to P14. Similarly, the expression also varies with age in the jejunum, where TRPV6 levels increases from P1 to P14, become weak at 1-month age and becomes undetectable in older mice.[112] The expression of TRPV6 in older rats (12-months) is at least 50% lower in comparison to younger counterparts (2-months old).[104] In both WT and VDR KO mice, the age-associated decline in intestinal absorption of Ca2+ is accompanied by a decline in duodenal expression of TRPV6.[113]
Sex hormones
Sex hormones play an important role in the regulation of TRPV6. In comparison to male mice, female mice exhibit a 2-fold higher increase in duodenal expression of TRPV6 mRNA following vitamin D treatment. Sex hormone-associated differential regulation of TRPV6 across genders is believed to be correlated to differences in relative risk to osteoporosis in older postmenopausal women which have been reported to have lower TRPV6 and VDR expression in comparison to males.[81]
Estrogen treatment upregulates TRPV6 transcripts by 8-fold in VDR KO mice and by 4-fold in ovariectomized mice.[106] Greater than 50% reduction in TRPV6 mRNA has been observed in estrogen receptor α KO mice.[110] It is believed that estrogen could be differentially regulating Ca2+ absorption in the duodenum by increasing TRPV6 expression through ERα.[114] Anti-progesterone agent RU486 and anti-estrogen agent ICI 182,780 suppress TRPV6 expression in rodents by their respective antagonist action on progesterone and estrogen receptors.[115] Estrogen, progesterone, and dexamethasone are known to upregulate TRPV6 expression in the cerebral cortex and hypothalamus of mice suggesting a potential involvement of TRPV6 in calcium absorption in the brain.[116]
Glucocorticoids
Subcutaneous administration of glucocorticoids dexamethasone induces both renal and intestinal expression of TRPV6 in mice within 24 hours of whereas oral application of prednisolone reduction in TRPV6 which is also accompanied by reduced Ca2+ absorption in duodenum.[117][118] Intestinal regulation of TRPV6 in response to glucocorticoids appears to be VDR-dependent.[117][118] The enzyme serum and glucocorticoid-regulated kinase 1 (SKG1) regulates TRPV6 expression by enhancing phosphatidylinositol-3-phosphate-5-kinase PIKfyve (PIP5K3).[119] This kinase is critical for the generation of secondary messenger PIP2, a known lipid activator of TRPV6.[119]
- TRPV
- TRPV5
- calcium channels
- calcium absorption
- transcellular pathway
- gating mechanism
- calmodulin
- maternal-fetal transport
- transient neonatal hyperparathyroidism
- chronic pancreatitis
- kidney stones
- cancer
Notes
References
- 1 2 3 ENSG00000165125 GRCh38: Ensembl release 89: ENSG00000276971, ENSG00000165125 - Ensembl, May 2017
- 1 2 3 GRCm38: Ensembl release 89: ENSMUSG00000029868 - Ensembl, May 2017
- ↑ "Human PubMed Reference:". National Center for Biotechnology Information, U.S. National Library of Medicine.
- ↑ "Mouse PubMed Reference:". National Center for Biotechnology Information, U.S. National Library of Medicine.
- 1 2 3 Venkatachalam K, Montell C (2007). "TRP channels". Annual Review of Biochemistry. 76: 387–417. doi:10.1146/annurev.biochem.75.103004.142819. PMC 4196875. PMID 17579562.
- ↑ Montell C, Birnbaumer L, Flockerzi V, Bindels RJ, Bruford EA, Caterina MJ, et al. (February 2002). "A unified nomenclature for the superfamily of TRP cation channels". Molecular Cell. 9 (2): 229–31. doi:10.1016/s1097-2765(02)00448-3. OCLC 1106536919. PMID 11864597.
- 1 2 Yue L, Peng JB, Hediger MA, Clapham DE (April 2001). "CaT1 manifests the pore properties of the calcium-release-activated calcium channel". Nature. 410 (6829): 705–9. Bibcode:2001Natur.410..705Y. doi:10.1038/35070596. PMID 11287959. S2CID 4404582.
- 1 2 3 4 5 Yelshanskaya MV, Nadezhdin KD, Kurnikova MG, Sobolevsky AI (February 2020). "Structure and function of the calcium-selective TRP channel TRPV6". The Journal of Physiology. 599 (10): 2673–2697. doi:10.1113/JP279024. PMC 7689878. PMID 32073143.
- 1 2 3 4 Peng JB, Suzuki Y, Gyimesi G, Hediger MA (2018). "TRPV5 and TRPV6 Calcium-Selective Channels.". Calcium Entry Channels in Non-Excitable Cells. Methods in signal transduction series. Boca Raton, FL: CRC Press/Taylor & Francis. pp. 241–274. doi:10.1201/9781315152592-13. ISBN 978-1-315-15259-2. PMID 30299660.
- 1 2 3 4 Peng JB, Chen XZ, Berger UV, Vassilev PM, Tsukaguchi H, Brown EM, Hediger MA (August 1999). "Molecular cloning and characterization of a channel-like transporter mediating intestinal calcium absorption". The Journal of Biological Chemistry. 274 (32): 22739–46. doi:10.1074/jbc.274.32.22739. PMID 10428857. S2CID 23616713.
- 1 2 Peng JB, Chen XZ, Berger UV, Weremowicz S, Morton CC, Vassilev PM, et al. (November 2000). "Human calcium transport protein CaT1". Biochemical and Biophysical Research Communications. 278 (2): 326–32. doi:10.1006/bbrc.2000.3716. PMID 11097838.
- 1 2 3 Weber K, Erben RG, Rump A, Adamski J (December 2001). "Gene structure and regulation of the murine epithelial calcium channels ECaC1 and 2". Biochemical and Biophysical Research Communications. 289 (5): 1287–94. doi:10.1006/bbrc.2001.6121. PMID 11741335.
- 1 2 3 Hoenderop JG, Vennekens R, Müller D, Prenen J, Droogmans G, Bindels RJ, Nilius B (December 2001). "Function and expression of the epithelial Ca(2+) channel family: comparison of mammalian ECaC1 and 2". The Journal of Physiology. 537 (Pt 3): 747–61. doi:10.1113/jphysiol.2001.012917. PMC 2278984. PMID 11744752.
- 1 2 3 4 5 6 7 8 Wissenbach U, Niemeyer BA, Fixemer T, Schneidewind A, Trost C, Cavalie A, et al. (June 2001). "Expression of CaT-like, a novel calcium-selective channel, correlates with the malignancy of prostate cancer". The Journal of Biological Chemistry. 276 (22): 19461–8. doi:10.1074/jbc.m009895200. PMID 11278579. S2CID 25833991.
- ↑ Clapham DE, Julius D, Montell C, Schultz G (December 2005). "International Union of Pharmacology. XLIX. Nomenclature and structure-function relationships of transient receptor potential channels". Pharmacological Reviews. 57 (4): 427–50. doi:10.1124/pr.57.4.6. PMID 16382100. S2CID 17936350.
- 1 2 Müller D, Hoenderop JG, Merkx GF, van Os CH, Bindels RJ (August 2000). "Gene structure and chromosomal mapping of human epithelial calcium channel". Biochemical and Biophysical Research Communications. 275 (1): 47–52. doi:10.1006/bbrc.2000.3227. PMID 10944439.
- 1 2 3 Fecher-Trost C, Weissgerber P, Wissenbach U (2014). "TRPV6 Channels". Mammalian Transient Receptor Potential (TRP) Cation Channels. Handbook of Experimental Pharmacology. Vol. 222. Berlin, Heidelberg: Springer Berlin Heidelberg. pp. 359–84. doi:10.1007/978-3-642-54215-2_14. ISBN 978-3-642-54214-5. PMID 24756713.
- 1 2 Fecher-Trost C, Wissenbach U, Beck A, Schalkowsky P, Stoerger C, Doerr J, et al. (June 2013). "The in vivo TRPV6 protein starts at a non-AUG triplet, decoded as methionine, upstream of canonical initiation at AUG". The Journal of Biological Chemistry. 288 (23): 16629–44. doi:10.1074/jbc.M113.469726. PMC 3675598. PMID 23612980.
- 1 2 3 Flores-Aldama L, Vandewege MW, Zavala K, Colenso CK, Gonzalez W, Brauchi SE, Opazo JC (May 2020). "Evolutionary analyses reveal independent origins of gene repertoires and structural motifs associated to fast inactivation in calcium-selective TRPV channels". Scientific Reports. 10 (1): 8684. Bibcode:2020NatSR..10.8684F. doi:10.1038/s41598-020-65679-6. PMC 7250927. PMID 32457384.
- 1 2 3 4 5 6 Akey JM, Swanson WJ, Madeoy J, Eberle M, Shriver MD (July 2006). "TRPV6 exhibits unusual patterns of polymorphism and divergence in worldwide populations". Human Molecular Genetics. 15 (13): 2106–13. doi:10.1093/hmg/ddl134. PMID 16717058.
- 1 2 3 Soejima M, Tachida H, Koda Y (February 2009). "Sequence analysis of human TRPV6 suggests positive selection outside Africa". Biochemical Genetics. 47 (1–2): 147–53. doi:10.1007/s10528-009-9222-x. PMID 19169858. S2CID 39897282.
- 1 2 3 4 5 Peng JB (2011). "TRPV5 and TRPV6 in Transcellular Ca2+ Transport: Regulation, Gene Duplication, and Polymorphisms in African Populations". Transient Receptor Potential Channels. Advances in Experimental Medicine and Biology. Vol. 704. pp. 239–75. doi:10.1007/978-94-007-0265-3_14. ISBN 978-94-007-0264-6. PMID 21290300.
- 1 2 3 4 Zhuang L, Peng JB, Tou L, Takanaga H, Adam RM, Hediger MA, Freeman MR (December 2002). "Calcium-selective ion channel, CaT1, is apically localized in gastrointestinal tract epithelia and is aberrantly expressed in human malignancies". Laboratory Investigation; A Journal of Technical Methods and Pathology. 82 (12): 1755–64. doi:10.1097/01.lab.0000043910.41414.e7. PMID 12480925. S2CID 6053178.
- 1 2 "TRPV6 protein expression summary". The Human Protein Atlas. Retrieved 2020-08-01.
- 1 2 Lehen'kyi V, Raphaël M, Prevarskaya N (March 2012). "The role of the TRPV6 channel in cancer". The Journal of Physiology. 590 (6): 1369–76. doi:10.1113/jphysiol.2011.225862. PMC 3382328. PMID 22331416.
- ↑ Hirnet D, Olausson J, Fecher-Trost C, Bödding M, Nastainczyk W, Wissenbach U, et al. (May 2003). "The TRPV6 gene, cDNA and protein". Cell Calcium. 33 (5–6): 509–18. doi:10.1016/s0143-4160(03)00066-6. PMID 12765696.
- ↑ Nijenhuis T, Hoenderop JG, van der Kemp AW, Bindels RJ (November 2003). "Localization and regulation of the epithelial Ca2+ channel TRPV6 in the kidney". Journal of the American Society of Nephrology. 14 (11): 2731–40. doi:10.1097/01.asn.0000094081.78893.e8. PMID 14569082.
- 1 2 3 4 5 Stewart JM (2020). "TRPV6 as A Target for Cancer Therapy". Journal of Cancer. 11 (2): 374–387. doi:10.7150/jca.31640. PMC 6930427. PMID 31897233.
- 1 2 Chen F, Ni B, Yang YO, Ye T, Chen A (2014). "Knockout of TRPV6 causes osteopenia in mice by increasing osteoclastic differentiation and activity". Cellular Physiology and Biochemistry. 33 (3): 796–809. doi:10.1159/000358653. PMID 24686448. S2CID 19539099.
- ↑ van der Eerden BC, Weissgerber P, Fratzl-Zelman N, Olausson J, Hoenderop JG, Schreuders-Koedam M, et al. (May 2012). "The transient receptor potential channel TRPV6 is dynamically expressed in bone cells but is not crucial for bone mineralization in mice". Journal of Cellular Physiology. 227 (5): 1951–9. doi:10.1002/jcp.22923. PMID 21732366. S2CID 7094759.
- ↑ Little R, Muimo R, Robson L, Harris K, Grabowski PS (2011-11-29). "The transient receptor potential ion channel TRPV6 is expressed at low levels in osteoblasts and has little role in osteoblast calcium uptake". PLOS ONE. 6 (11): e28166. Bibcode:2011PLoSO...628166L. doi:10.1371/journal.pone.0028166. PMC 3226639. PMID 22163264.
- 1 2 3 4 5 6 7 8 9 10 11 12 13 14 Saotome K, Singh AK, Yelshanskaya MV, Sobolevsky AI (June 2016). "Crystal structure of the epithelial calcium channel TRPV6". Nature. 534 (7608): 506–11. Bibcode:2016Natur.534..506S. doi:10.1038/nature17975. PMC 4919205. PMID 27296226.
- ↑ Singh AK, Saotome K, Sobolevsky AI (September 2017). "Swapping of transmembrane domains in the epithelial calcium channel TRPV6". Scientific Reports. 7 (1): 10669. Bibcode:2017NatSR...710669S. doi:10.1038/s41598-017-10993-9. PMC 5587609. PMID 28878326.
- 1 2 Lu P, Boros S, Chang Q, Bindels RJ, Hoenderop JG (November 2008). "The beta-glucuronidase klotho exclusively activates the epithelial Ca2+ channels TRPV5 and TRPV6". Nephrology, Dialysis, Transplantation. 23 (11): 3397–402. doi:10.1093/ndt/gfn291. hdl:2066/70822. PMID 18495742.
- 1 2 3 Owsianik G, Talavera K, Voets T, Nilius B (January 2006). "Permeation and selectivity of TRP channels". Annual Review of Physiology. 68 (1): 685–717. doi:10.1146/annurev.physiol.68.040204.101406. PMID 16460288.
- 1 2 3 Voets T, Janssens A, Droogmans G, Nilius B (April 2004). "Outer pore architecture of a Ca2+-selective TRP channel". The Journal of Biological Chemistry. 279 (15): 15223–30. doi:10.1074/jbc.m312076200. PMID 14736889. S2CID 23827272.
- ↑ Voets T, Janssens A, Prenen J, Droogmans G, Nilius B (March 2003). "Mg2+-dependent gating and strong inward rectification of the cation channel TRPV6". The Journal of General Physiology. 121 (3): 245–60. doi:10.1085/jgp.20028752. PMC 2217333. PMID 12601087.
- ↑ Kovacs G, Danko T, Bergeron MJ, Balazs B, Suzuki Y, Zsembery A, Hediger MA (January 2011). "Heavy metal cations permeate the TRPV6 epithelial cation channel". Cell Calcium. 49 (1): 43–55. doi:10.1016/j.ceca.2010.11.007. PMID 21146870. S2CID 41086787.
- 1 2 3 4 5 Singh AK, Saotome K, Sobolevsky AI (September 2017). "Swapping of transmembrane domains in the epithelial calcium channel TRPV6". Scientific Reports. 7 (1): 10669. Bibcode:2017NatSR...710669S. bioRxiv 10.1101/141523. doi:10.1038/s41598-017-10993-9. PMC 5587609. PMID 28878326.
- 1 2 3 4 Cao C, Zakharian E, Borbiro I, Rohacs T (February 2013). "Interplay between calmodulin and phosphatidylinositol 4,5-bisphosphate in Ca2+-induced inactivation of transient receptor potential vanilloid 6 channels". The Journal of Biological Chemistry. 288 (8): 5278–90. doi:10.1074/jbc.M112.409482. PMC 3581402. PMID 23300090.
- 1 2 3 Bödding M, Flockerzi V (August 2004). "Ca2+ dependence of the Ca2+-selective TRPV6 channel". The Journal of Biological Chemistry. 279 (35): 36546–52. doi:10.1074/jbc.m404679200. PMID 15184369. S2CID 22842694.
- 1 2 Lambers TT, Weidema AF, Nilius B, Hoenderop JG, Bindels RJ (July 2004). "Regulation of the mouse epithelial Ca2(+) channel TRPV6 by the Ca(2+)-sensor calmodulin". The Journal of Biological Chemistry. 279 (28): 28855–61. doi:10.1074/jbc.m313637200. hdl:2066/57723. PMID 15123711. S2CID 23453339.
- 1 2 3 Zakharian E, Cao C, Rohacs T (November 2011). "Intracellular ATP supports TRPV6 activity via lipid kinases and the generation of PtdIns(4,5) P₂". FASEB Journal. 25 (11): 3915–28. doi:10.1096/fj.11-184630. PMC 3205842. PMID 21810903.
- 1 2 3 Thyagarajan B, Benn BS, Christakos S, Rohacs T (March 2009). "Phospholipase C-mediated regulation of transient receptor potential vanilloid 6 channels: implications in active intestinal Ca2+ transport". Molecular Pharmacology. 75 (3): 608–16. doi:10.1124/mol.108.052449. PMC 2684912. PMID 19073818.
- ↑ Derler I, Hofbauer M, Kahr H, Fritsch R, Muik M, Kepplinger K, et al. (November 2006). "Dynamic but not constitutive association of calmodulin with rat TRPV6 channels enables fine tuning of Ca2+-dependent inactivation". The Journal of Physiology. 577 (Pt 1): 31–44. doi:10.1113/jphysiol.2006.118661. PMC 2000671. PMID 16959851.
- ↑ Niemeyer BA, Bergs C, Wissenbach U, Flockerzi V, Trost C (March 2001). "Competitive regulation of CaT-like-mediated Ca2+ entry by protein kinase C and calmodulin". Proceedings of the National Academy of Sciences of the United States of America. 98 (6): 3600–5. Bibcode:2001PNAS...98.3600N. doi:10.1073/pnas.051511398. PMC 30699. PMID 11248124.
- ↑ Shin YC, Shin SY, So I, Kwon D, Jeon JH (January 2011). "TRIP Database: a manually curated database of protein-protein interactions for mammalian TRP channels". Nucleic Acids Research. 39 (Database issue): D356-61. doi:10.1093/nar/gkq814. PMC 3013757. PMID 20851834. S2CID 16278877.
- 1 2 Suzuki Y, Landowski CP, Hediger MA (March 2008). "Mechanisms and regulation of epithelial Ca2+ absorption in health and disease". Annual Review of Physiology. 70 (1): 257–71. doi:10.1146/annurev.physiol.69.031905.161003. PMID 17850211.
- 1 2 3 4 5 6 Suzuki Y, Kovacs CS, Takanaga H, Peng JB, Landowski CP, Hediger MA (August 2008). "Calcium channel TRPV6 is involved in murine maternal-fetal calcium transport". Journal of Bone and Mineral Research. 23 (8): 1249–56. doi:10.1359/jbmr.080314. PMC 2680174. PMID 18348695.
- 1 2 Lehen'kyi V, Beck B, Polakowska R, Charveron M, Bordat P, Skryma R, Prevarskaya N (August 2007). "TRPV6 is a Ca2+ entry channel essential for Ca2+-induced differentiation of human keratinocytes". The Journal of Biological Chemistry. 282 (31): 22582–91. doi:10.1074/jbc.m611398200. PMID 17550901. S2CID 22082147.
- 1 2 3 Yamauchi D, Nakaya K, Raveendran NN, Harbidge DG, Singh R, Wangemann P, Marcus DC (January 2010). "Expression of epithelial calcium transport system in rat cochlea and vestibular labyrinth". BMC Physiology. 10 (1): 1. doi:10.1186/1472-6793-10-1. PMC 2825184. PMID 20113508. S2CID 5773117.
- 1 2 3 Yamauchi D, Raveendran NN, Pondugula SR, Kampalli SB, Sanneman JD, Harbidge DG, Marcus DC (June 2005). "Vitamin D upregulates expression of ECaC1 mRNA in semicircular canal". Biochemical and Biophysical Research Communications. 331 (4): 1353–7. doi:10.1016/j.bbrc.2005.04.053. PMID 15883024.
- 1 2 3 4 5 Weissgerber P, Kriebs U, Tsvilovskyy V, Olausson J, Kretz O, Stoerger C, et al. (May 2011). "Male fertility depends on Ca²+ absorption by TRPV6 in epididymal epithelia". Science Signaling. 4 (171): ra27. doi:10.1126/scisignal.2001791. PMID 21540454. S2CID 206670887.
- 1 2 3 Weissgerber P, Kriebs U, Tsvilovskyy V, Olausson J, Kretz O, Stoerger C, et al. (May 2012). "Excision of Trpv6 gene leads to severe defects in epididymal Ca2+ absorption and male fertility much like single D541A pore mutation". The Journal of Biological Chemistry. 287 (22): 17930–41. doi:10.1074/jbc.m111.328286. PMC 3365704. PMID 22427671.
- 1 2 3 Diaz de Barboza G, Guizzardi S, Tolosa de Talamoni N (June 2015). "Molecular aspects of intestinal calcium absorption". World Journal of Gastroenterology. 21 (23): 7142–54. doi:10.3748/wjg.v21.i23.7142. PMC 4476875. PMID 26109800.
- 1 2 3 4 Bianco SD, Peng JB, Takanaga H, Suzuki Y, Crescenzi A, Kos CH, et al. (February 2007). "Marked disturbance of calcium homeostasis in mice with targeted disruption of the Trpv6 calcium channel gene". Journal of Bone and Mineral Research. 22 (2): 274–85. doi:10.1359/jbmr.061110. PMC 4548943. PMID 17129178.
- ↑ Azarpeykan S, Dittmer KE, Marshall JC, Perera KC, Gee EK, Acke E, Thompson KG (2016-09-15). "Evaluation and Comparison of Vitamin D Responsive Gene Expression in Ovine, Canine and Equine Kidney". PLOS ONE. 11 (9): e0162598. Bibcode:2016PLoSO..1162598A. doi:10.1371/journal.pone.0162598. PMC 5025205. PMID 27632366.
- ↑ Stulc J (July 1997). "Placental transfer of inorganic ions and water". Physiological Reviews. 77 (3): 805–36. doi:10.1152/physrev.1997.77.3.805. PMID 9234966.
- ↑ Kovacs CS, Kronenberg HM (December 1997). "Maternal-fetal calcium and bone metabolism during pregnancy, puerperium, and lactation". Endocrine Reviews. 18 (6): 832–72. doi:10.1210/edrv.18.6.0319. PMID 9408745.
- ↑ Sharma D, Shastri S, Sharma P (January 2016). "Intrauterine Growth Restriction: Antenatal and Postnatal Aspects". Clinical Medicine Insights. Pediatrics. 10: 67–83. doi:10.4137/cmped.s40070. PMC 4946587. PMID 27441006.
- 1 2 Bernucci L, Henríquez M, Díaz P, Riquelme G (November 2006). "Diverse calcium channel types are present in the human placental syncytiotrophoblast basal membrane". Placenta. 27 (11–12): 1082–95. doi:10.1016/j.placenta.2005.12.007. hdl:10533/178038. PMID 16564089.
- ↑ Lee GS, Jeung EB (July 2007). "Uterine TRPV6 expression during the estrous cycle and pregnancy in a mouse model". American Journal of Physiology. Endocrinology and Metabolism. 293 (1): E132-8. doi:10.1152/ajpendo.00666.2006. PMID 17374692.
- ↑ Moreau R, Hamel A, Daoud G, Simoneau L, Lafond J (November 2002). "Expression of calcium channels along the differentiation of cultured trophoblast cells from human term placenta". Biology of Reproduction. 67 (5): 1473–9. doi:10.1095/biolreprod.102.005397. PMID 12390878. S2CID 23847059.
- ↑ Yang H, Kim TH, An BS, Choi KC, Lee HH, Kim JM, Jeung EB (March 2013). "Differential expression of calcium transport channels in placenta primary cells and tissues derived from preeclamptic placenta". Molecular and Cellular Endocrinology. 367 (1–2): 21–30. doi:10.1016/j.mce.2012.12.012. PMID 23267838. S2CID 5276054.
- ↑ Miura S, Sato K, Kato-Negishi M, Teshima T, Takeuchi S (November 2015). "Fluid shear triggers microvilli formation via mechanosensitive activation of TRPV6". Nature Communications. 6 (1): 8871. Bibcode:2015NatCo...6.8871M. doi:10.1038/ncomms9871. PMC 4660203. PMID 26563429.
- ↑ Ecroyd H, Asquith KL, Jones RC, Aitken RJ (April 2004). "The development of signal transduction pathways during epididymal maturation is calcium dependent". Developmental Biology. 268 (1): 53–63. doi:10.1016/j.ydbio.2003.12.015. PMID 15031104.
- ↑ Gao D, Zhang BL, Leung MC, Au SC, Wong PY, Shum WW (August 2016). "Coupling of TRPV6 and TMEM16A in epithelial principal cells of the rat epididymis". The Journal of General Physiology. 148 (2): 161–82. doi:10.1085/jgp.201611626. PMC 4969799. PMID 27481714.
- 1 2 3 4 Lieben L, Benn BS, Ajibade D, Stockmans I, Moermans K, Hediger MA, et al. (August 2010). "Trpv6 mediates intestinal calcium absorption during calcium restriction and contributes to bone homeostasis". Bone. 47 (2): 301–8. doi:10.1016/j.bone.2010.04.595. PMC 2902603. PMID 20399919.
- 1 2 3 Lieben L, Carmeliet G (2012). "The Involvement of TRP Channels in Bone Homeostasis". Frontiers in Endocrinology. 3: 99. doi:10.3389/fendo.2012.00099. PMC 3422722. PMID 22934090.
- ↑ Tu CL, Bikle DD (June 2013). "Role of the calcium-sensing receptor in calcium regulation of epidermal differentiation and function". Best Practice & Research. Clinical Endocrinology & Metabolism. 27 (3): 415–27. doi:10.1016/j.beem.2013.03.002. PMC 3713412. PMID 23856269.
- 1 2 3 4 Nakaya K, Harbidge DG, Wangemann P, Schultz BD, Green ED, Wall SM, Marcus DC (May 2007). "Lack of pendrin HCO3- transport elevates vestibular endolymphatic [Ca2+] by inhibition of acid-sensitive TRPV5 and TRPV6 channels". American Journal of Physiology. Renal Physiology. 292 (5): F1314-21. doi:10.1152/ajprenal.00432.2006. PMC 2515270. PMID 17200157.
- 1 2 De Clercq K, Held K, Van Bree R, Meuleman C, Peeraer K, Tomassetti C, et al. (June 2015). "Functional expression of transient receptor potential channels in human endometrial stromal cells during the luteal phase of the menstrual cycle". Human Reproduction. 30 (6): 1421–36. doi:10.1093/humrep/dev068. PMID 25820697.
- 1 2 3 Yang H, Choi KC, Hyun SH, Jeung EB (April 2011). "Coexpression and estrogen-mediated regulation of TRPV6 and PMCA1 in the human endometrium during the menstrual cycle". Molecular Reproduction and Development. 78 (4): 274–82. doi:10.1002/mrd.21303. PMID 21400627. S2CID 21140465.
- 1 2 De Clercq K, Van den Eynde C, Hennes A, Van Bree R, Voets T, Vriens J (March 2017). "The functional expression of transient receptor potential channels in the mouse endometrium". Human Reproduction. 32 (3): 615–630. doi:10.1093/humrep/dew344. PMID 28077439. S2CID 3409475.
- ↑ Suzuki Y, Chitayat D, Sawada H, Deardorff MA, McLaughlin HM, Begtrup A, Millar K, Harrington J, Chong K, Roifman M, Grand K, Tominaga M, Takada F, Shuster S, Obara M, Mutoh H, Kushima R, Nishimura G (June 2018). "TRPV6 Variants Interfere with Maternal-Fetal Calcium Transport through the Placenta and Cause Transient Neonatal Hyperparathyroidism". American Journal of Human Genetics. 102 (6): 1104–1114. doi:10.1016/j.ajhg.2018.04.006. PMC 5992228. PMID 29861107.
- ↑ Yamashita S, Mizumoto H, Sawada H, Suzuki Y, Hata D (March 2019). "TRPV6 Gene Mutation in a Dizygous Twin With Transient Neonatal Hyperparathyroidism". Journal of the Endocrine Society. 3 (3): 602–606. doi:10.1210/js.2018-00374. PMC 6389352. PMID 30820485.
- ↑ Burren CP, Caswell R, Castle B, Welch CR, Hilliard TN, Smithson SF, Ellard S (September 2018). "TRPV6 compound heterozygous variants result in impaired placental calcium transport and severe undermineralization and dysplasia of the fetal skeleton". American Journal of Medical Genetics. Part A. 176 (9): 1950–1955. doi:10.1002/ajmg.a.40484. PMC 6563443. PMID 30144375.
- 1 2 Zou WB, Wang YC, Ren XL, Wang L, Deng SJ, Mao XT, et al. (August 2020). "TRPV6 variants confer susceptibility to chronic pancreatitis in the Chinese population". Human Mutation. 41 (8): 1351–1357. doi:10.1002/humu.24032. PMID 32383311.
- 1 2 3 4 Masamune A, Kotani H, Sörgel FL, Chen JM, Hamada S, Sakaguchi R, et al. (May 2020). "Variants That Affect Function of Calcium Channel TRPV6 Are Associated With Early-Onset Chronic Pancreatitis". Gastroenterology. 158 (6): 1626–1641.e8. doi:10.1053/j.gastro.2020.01.005. PMID 31930989.
- 1 2 Suzuki, Yoshiro Pasch, Andreas Bonny, Olivier Mohaupt, Markus G. Hediger, Matthias A. Frey, Felix J. (2017-08-02). Gain-of-function haplotype in the epithelial calcium channel TRPV6 is a risk factor for renal calcium stone formation. Vol. 17. pp. 1613–8. doi:10.1093/hmg/ddn048. OCLC 1156692319. PMID 18276610.
{{cite book}}
:|journal=
ignored (help)CS1 maint: multiple names: authors list (link) - 1 2 Walters JR, Balesaria S, Chavele KM, Taylor V, Berry JL, Khair U, et al. (November 2006). "Calcium channel TRPV6 expression in human duodenum: different relationships to the vitamin D system and aging in men and women". Journal of Bone and Mineral Research. 21 (11): 1770–7. doi:10.1359/jbmr.060721. PMID 17002582. S2CID 22847166.
- 1 2 Sherwood J, Bertrand J, Seidemann M, Dell'Accio F, Pap T (April 2016). "Activation of the transient receptor potential cation channel TRPC6 is required for chondrocyte phenotypic stability". Osteoarthritis and Cartilage. 24: S152–S153. doi:10.1016/j.joca.2016.01.298. ISSN 1063-4584.
- 1 2 3 4 Dror AA, Brownstein Z, Avraham KB (2011). "Integration of human and mouse genetics reveals pendrin function in hearing and deafness". Cellular Physiology and Biochemistry. 28 (3): 535–44. doi:10.1159/000335163. PMC 3709173. PMID 22116368.
- ↑ Sun F, Xiao L, Jang XX, Xiong Y, Li Q, Yue XJ, et al. (October 2016). "TRPV6 is a prognostic marker in early-stage cervical squamous cell carcinoma". Tumour Biology. 37 (12): 15743–15751. doi:10.1007/s13277-016-5368-4. PMID 27747588. S2CID 6445506.
- 1 2 Fan H, Shen YX, Yuan YF (2014-03-30). "Expression and prognostic roles of TRPV5 and TRPV6 in non-small cell lung cancer after curative resection". Asian Pacific Journal of Cancer Prevention. 15 (6): 2559–63. doi:10.7314/apjcp.2014.15.6.2559. PMID 24761864.
- ↑ Wu Y, Miyamoto T, Li K, Nakagomi H, Sawada N, Kira S, et al. (December 2011). "Decreased expression of the epithelial Ca2+ channel TRPV5 and TRPV6 in human renal cell carcinoma associated with vitamin D receptor". The Journal of Urology. 186 (6): 2419–25. doi:10.1016/j.juro.2011.07.086. PMID 22019165.
- 1 2 3 4 5 Peng JB, Zhuang L, Berger UV, Adam RM, Williams BJ, Brown EM, et al. (April 2001). "CaT1 expression correlates with tumor grade in prostate cancer". Biochemical and Biophysical Research Communications. 282 (3): 729–34. doi:10.1006/bbrc.2001.4638. PMID 11401523.
- 1 2 Fixemer T, Wissenbach U, Flockerzi V, Bonkhoff H (October 2003). "Expression of the Ca2+-selective cation channel TRPV6 in human prostate cancer: a novel prognostic marker for tumor progression". Oncogene. 22 (49): 7858–61. doi:10.1038/sj.onc.1206895. PMID 14586412. S2CID 23626142.
- ↑ Dhennin-Duthille I, Gautier M, Faouzi M, Guilbert A, Brevet M, Vaudry D, et al. (2011). "High expression of transient receptor potential channels in human breast cancer epithelial cells and tissues: correlation with pathological parameters". Cellular Physiology and Biochemistry. 28 (5): 813–22. doi:10.1159/000335795. PMID 22178934. S2CID 45740662.
- 1 2 Bolanz KA, Hediger MA, Landowski CP (February 2008). "The role of TRPV6 in breast carcinogenesis". Molecular Cancer Therapeutics. 7 (2): 271–9. doi:10.1158/1535-7163.mct-07-0478. PMID 18245667. S2CID 17158946.
- ↑ Peters AA, Simpson PT, Bassett JJ, Lee JM, Da Silva L, Reid LE, et al. (October 2012). "Calcium channel TRPV6 as a potential therapeutic target in estrogen receptor-negative breast cancer". Molecular Cancer Therapeutics. 11 (10): 2158–68. doi:10.1158/1535-7163.mct-11-0965. PMID 22807578. S2CID 207614323.
- 1 2 3 Lehen'kyi V, Flourakis M, Skryma R, Prevarskaya N (November 2007). "TRPV6 channel controls prostate cancer cell proliferation via Ca(2+)/NFAT-dependent pathways". Oncogene. 26 (52): 7380–5. doi:10.1038/sj.onc.1210545. PMID 17533368. S2CID 482760.
- ↑ Lehen'kyi V, Raphaël M, Oulidi A, Flourakis M, Khalimonchyk S, Kondratskyi A, et al. (February 2011). "TRPV6 determines the effect of vitamin D3 on prostate cancer cell growth". PLOS ONE. 6 (2): e16856. Bibcode:2011PLoSO...616856L. doi:10.1371/journal.pone.0016856. PMC 3037935. PMID 21347289.
- ↑ Bolanz KA, Kovacs GG, Landowski CP, Hediger MA (December 2009). "Tamoxifen inhibits TRPV6 activity via estrogen receptor-independent pathways in TRPV6-expressing MCF-7 breast cancer cells". Molecular Cancer Research. 7 (12): 2000–10. doi:10.1158/1541-7786.mcr-09-0188. PMID 19996302. S2CID 26337876.
- ↑ Peleg S, Sellin JH, Wang Y, Freeman MR, Umar S (September 2010). "Suppression of aberrant transient receptor potential cation channel, subfamily V, member 6 expression in hyperproliferative colonic crypts by dietary calcium". American Journal of Physiology. Gastrointestinal and Liver Physiology. 299 (3): G593-601. doi:10.1152/ajpgi.00193.2010. PMC 2950683. PMID 20508153.
- ↑ Arbabian A, Iftinca M, Altier C, Singh PP, Isambert H, Coscoy S (December 2020). "Mutations in calmodulin-binding domains of TRPV4/6 channels confer invasive properties to colon adenocarcinoma cells". Channels. 14 (1): 101–109. doi:10.1080/19336950.2020.1740506. PMC 7153789. PMID 32186440.
- ↑ Ishizawa M, Akagi D, Yamamoto J, Makishima M (September 2017). "3 enhances TRPV6 transcription through p38 MAPK activation and GADD45 expression". The Journal of Steroid Biochemistry and Molecular Biology. 172: 55–61. doi:10.1016/j.jsbmb.2017.05.013. PMID 28578001. S2CID 206502344.
- ↑ Dai W, Bai Y, Hebda L, Zhong X, Liu J, Kao J, Duan C (April 2014). "Calcium deficiency-induced and TRP channel-regulated IGF1R-PI3K-Akt signaling regulates abnormal epithelial cell proliferation". Cell Death and Differentiation. 21 (4): 568–81. doi:10.1038/cdd.2013.177. PMC 3950320. PMID 24336047.
- ↑ Skrzypski M, Kołodziejski PA, Mergler S, Khajavi N, Nowak KW, Strowski MZ (August 2016). "TRPV6 modulates proliferation of human pancreatic neuroendocrine BON-1 tumour cells". Bioscience Reports. 36 (4). doi:10.1042/bsr20160106. PMC 4995500. PMID 27450545.
- ↑ Song H, Dong M, Zhou J, Sheng W, Li X, Gao W (March 2018). "Expression and prognostic significance of TRPV6 in the development and progression of pancreatic cancer". Oncology Reports. 39 (3): 1432–1440. doi:10.3892/or.2018.6216. PMID 29344675.
- ↑ Chow J, Norng M, Zhang J, Chai J (April 2007). "TRPV6 mediates capsaicin-induced apoptosis in gastric cancer cells--Mechanisms behind a possible new "hot" cancer treatment". Biochimica et Biophysica Acta (BBA) - Molecular Cell Research. 1773 (4): 565–76. doi:10.1016/j.bbamcr.2007.01.001. PMID 17292493.
- ↑ Zhang SS, Xie X, Wen J, Luo KJ, Liu QW, Yang H, et al. (January 2016). "TRPV6 plays a new role in predicting survival of patients with esophageal squamous cell carcinoma". Diagnostic Pathology. 11 (1): 14. doi:10.1186/s13000-016-0457-7. PMC 4730645. PMID 26818094.
- 1 2 3 Song Y, Peng X, Porta A, Takanaga H, Peng JB, Hediger MA, et al. (September 2003). "Calcium transporter 1 and epithelial calcium channel messenger ribonucleic acid are differentially regulated by 1,25 dihydroxyvitamin D3 in the intestine and kidney of mice". Endocrinology. 144 (9): 3885–94. doi:10.1210/en.2003-0314. PMID 12933662.
- 1 2 3 4 5 6 Brown AJ, Krits I, Armbrecht HJ (May 2005). "Effect of age, vitamin D, and calcium on the regulation of rat intestinal epithelial calcium channels". Archives of Biochemistry and Biophysics. 437 (1): 51–8. doi:10.1016/j.abb.2005.02.007. PMID 15820216.
- 1 2 Meyer MB, Watanuki M, Kim S, Shevde NK, Pike JW (June 2006). "The human transient receptor potential vanilloid type 6 distal promoter contains multiple vitamin D receptor binding sites that mediate activation by 1,25-dihydroxyvitamin D3 in intestinal cells". Molecular Endocrinology. 20 (6): 1447–61. doi:10.1210/me.2006-0031. PMID 16574738.
- 1 2 Song Y, Kato S, Fleet JC (February 2003). "Vitamin D receptor (VDR) knockout mice reveal VDR-independent regulation of intestinal calcium absorption and ECaC2 and calbindin D9k mRNA". The Journal of Nutrition. 133 (2): 374–80. doi:10.1093/jn/133.2.374. PMID 12566470.
- ↑ Christakos S, Dhawan P, Verstuyf A, Verlinden L, Carmeliet G (January 2016). "Vitamin D: Metabolism, Molecular Mechanism of Action, and Pleiotropic Effects". Physiological Reviews. 96 (1): 365–408. doi:10.1152/physrev.00014.2015. PMC 4839493. PMID 26681795.
- ↑ Bouillon R, Van Cromphaut S, Carmeliet G (February 2003). "Intestinal calcium absorption: Molecular vitamin D mediated mechanisms". Journal of Cellular Biochemistry. 88 (2): 332–9. doi:10.1002/jcb.10360. PMID 12520535. S2CID 9853381.
- ↑ Replogle RA, Li Q, Wang L, Zhang M, Fleet JC (March 2014). "Gene-by-diet interactions influence calcium absorption and bone density in mice". Journal of Bone and Mineral Research. 29 (3): 657–65. doi:10.1002/jbmr.2065. PMC 10591522. PMID 23955923. S2CID 1546230.
- 1 2 Van Cromphaut SJ, Rummens K, Stockmans I, Van Herck E, Dijcks FA, Ederveen AG, et al. (October 2003). "Intestinal calcium transporter genes are upregulated by estrogens and the reproductive cycle through vitamin D receptor-independent mechanisms". Journal of Bone and Mineral Research. 18 (10): 1725–36. doi:10.1359/jbmr.2003.18.10.1725. PMID 14584880. S2CID 25346500.
- ↑ Charoenphandhu N, Nakkrasae LI, Kraidith K, Teerapornpuntakit J, Thongchote K, Thongon N, Krishnamra N (September 2009). "Two-step stimulation of intestinal Ca(2+) absorption during lactation by long-term prolactin exposure and suckling-induced prolactin surge". American Journal of Physiology. Endocrinology and Metabolism. 297 (3): E609-19. doi:10.1152/ajpendo.00347.2009. PMID 19567804.
- 1 2 Beggs MR, Lee JJ, Busch K, Raza A, Dimke H, Weissgerber P, et al. (2019). "v1.3 Mediate Distal Small Intestine Calcium Absorption Before Weaning". Cellular and Molecular Gastroenterology and Hepatology. 8 (4): 625–642. doi:10.1016/j.jcmgh.2019.07.005. PMC 6889763. PMID 31398491.
- ↑ van Abel M, Huybers S, Hoenderop JG, van der Kemp AW, van Leeuwen JP, Bindels RJ (December 2006). "Age-dependent alterations in Ca2+ homeostasis: role of TRPV5 and TRPV6". American Journal of Physiology. Renal Physiology. 291 (6): F1177-83. doi:10.1152/ajprenal.00038.2006. PMID 16705151.
- ↑ Nie X, Jin H, Wen G, Xu J, An J, Liu X, et al. (January 2020). "Estrogen Regulates Duodenal Calcium Absorption Through Differential Role of Estrogen Receptor on Calcium Transport Proteins". Digestive Diseases and Sciences. 65 (12): 3502–3513. doi:10.1007/s10620-020-06076-x. PMID 31974908. S2CID 210862678.
- ↑ Lee BM, Lee GS, Jung EM, Choi KC, Jeung EB (May 2009). "Uterine and placental expression of TRPV6 gene is regulated via progesterone receptor- or estrogen receptor-mediated pathways during pregnancy in rodents". Reproductive Biology and Endocrinology. 7 (1): 49. doi:10.1186/1477-7827-7-49. PMC 2694200. PMID 19457270.
- ↑ Park S, Yoo Y, Jung E, Jeung E (April 2020). "The effect of steroid hormone on the expression of the calcium-processing proteins in the immature female rat brain". Journal of Chemical Neuroanatomy. 105: 101767. doi:10.1016/j.jchemneu.2020.101767. PMID 32061997. S2CID 211105316.
- 1 2 Kim MH, Lee GS, Jung EM, Choi KC, Jeung EB (July 2009). "The negative effect of dexamethasone on calcium-processing gene expressions is associated with a glucocorticoid-induced calcium-absorbing disorder". Life Sciences. 85 (3–4): 146–52. doi:10.1016/j.lfs.2009.05.013. PMID 19490920.
- 1 2 Koo TH, Yang H, Jeung EB (2011-07-01). "Expression of Calcium Transport Genes in the Placenta of Calbindin-D9k and -D28k Knockout Mice". Biology of Reproduction. 85 (Suppl_1): 449. doi:10.1093/biolreprod/85.s1.449. ISSN 0006-3363.
- 1 2 Sopjani M, Kunert A, Czarkowski K, Klaus F, Laufer J, Föller M, Lang F (February 2010). "Regulation of the Ca(2+) channel TRPV6 by the kinases SGK1, PKB/Akt, and PIKfyve". The Journal of Membrane Biology. 233 (1–3): 35–41. doi:10.1007/s00232-009-9222-0. PMID 20041238. S2CID 25349984.
Further reading
- Heiner I, Eisfeld J, Lückhoff A (2004). "Role and regulation of TRP channels in neutrophil granulocytes". Cell Calcium. 33 (5–6): 533–40. doi:10.1016/S0143-4160(03)00058-7. PMID 12765698.
- Clapham DE, Julius D, Montell C, Schultz G (December 2005). "International Union of Pharmacology. XLIX. Nomenclature and structure-function relationships of transient receptor potential channels". Pharmacological Reviews. 57 (4): 427–50. doi:10.1124/pr.57.4.6. PMID 16382100. S2CID 17936350.
- Wissenbach U, Niemeyer BA (2007). "TRPV6". Transient Receptor Potential (TRP) Channels. Handbook of Experimental Pharmacology. Vol. 179. pp. 221–34. doi:10.1007/978-3-540-34891-7_13. ISBN 978-3-540-34889-4. PMID 17217060.
- Schoeber JP, Hoenderop JG, Bindels RJ (February 2007). "Concerted action of associated proteins in the regulation of TRPV5 and TRPV6". Biochemical Society Transactions. 35 (Pt 1): 115–9. doi:10.1042/BST0350115. PMID 17233615.
External links
- TRPV+Cation+Channels at the U.S. National Library of Medicine Medical Subject Headings (MeSH)
- TRPV6+protein,+human at the U.S. National Library of Medicine Medical Subject Headings (MeSH)